May 10, 2023PRESS RELEASE
Verifying quantum electrodynamics with exotic atoms
—Succeeding in precise measurements of muonic X-ray energy—
Keyword:RESEARCH
OBJECTIVE.
An international team of researchers used a superconducting transition-edge microcalorimeter (TES) [1], a state-of-the-art X-ray detector, to precisely measure the energy spectrum of muonic X-rays [3], which are emitted from muonic atoms [2] comprised of negative muons [2] and an atomic nucleus, in an investigation of strong-field quantum electrodynamics [4] with exotic atoms [5]. The team included Takuma Okumura, who at the time was a special researcher at RIKEN's Atomic, Molecular, and Optical Physics Laboratory and is currently an assistant professor in the Department of Chemistry at Tokyo Metropolitan University's Graduate School of Science; Toshiyuki Azuma, senior researcher at the Atomic, Molecular, and Optical Physics Laboratory; Tadashi Hashimoto, deputy research director at the Japan Atomic Energy Agency; Hideyuki Tatsuno, visiting researcher at Tokyo Metropolitan University; Shinya Yamada, associate professor at Rikkyo University; Paul Indelicato, professor at the Kasler-Brossell Institute; Tadayuki Takahashi, professor at the Kavli Institute for the Physics and Mathematics of the Universe at the University of Tokyo; Koichiro Shimomura, professor at the Institute of Materials Structure Science in the High Energy Accelerator Research Organization; and Shinji Okada, associate professor at Chubu University (now a full professor).
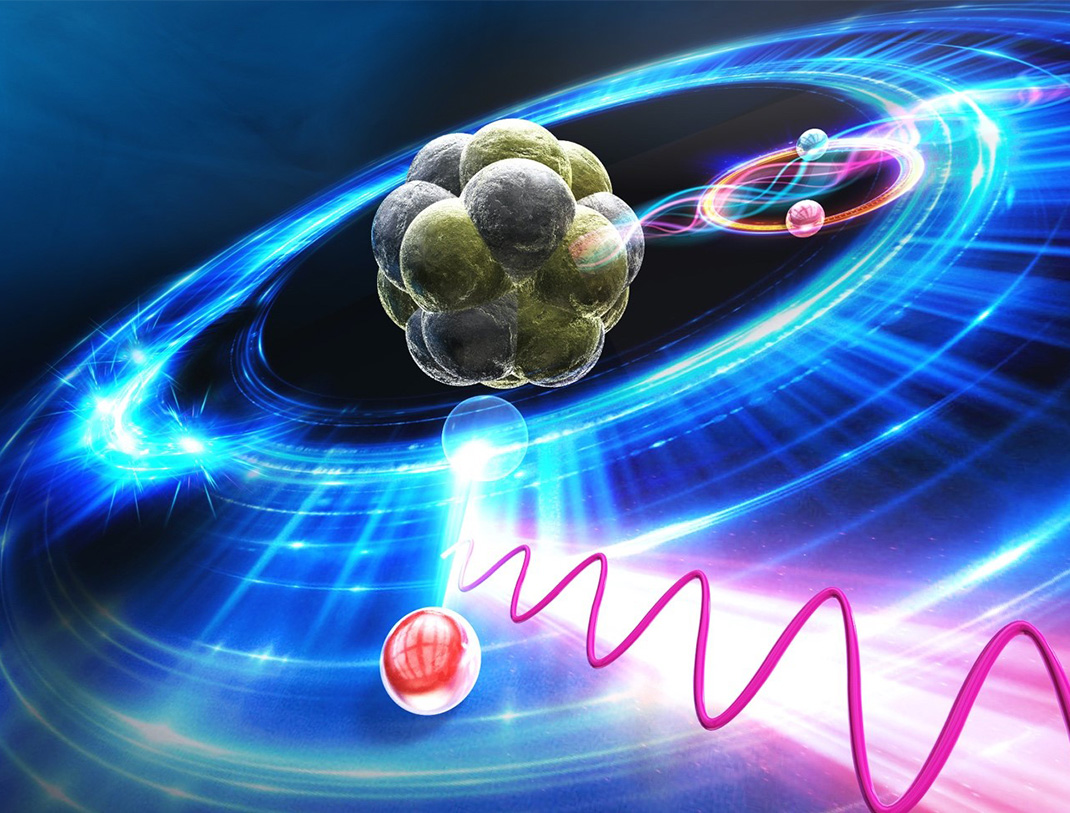
Conceptual diagram showing muonic neon atoms and quantum electromagnetic effects
The research group irradiated neon gas with a low-speed negative muon beam obtained from J-PARC's[7] high-intensity proton accelerator, and measured the muonic atoms (muonic neon atoms) this produced using the TES detector. By maximizing the high-energy resolution of the TES detector, the energy of the muonic X-rays could be determined at an absolute accuracy less than one 10,000th and the effect of vacuum polarization [8] in strong-field quantum electrodynamics at an extremely high accuracy of 5.8%.
Their findings were published online in the journal "Physical Review Letters" on April 27.
Background
The effects of QED are more pronounced in environments with strong electric fields, though this makes theoretical calculations more difficult. Therefore, a strong electric field environment is very important as a stage for QED experiments. For many years, polyvalent heavy ions, which are heavy atoms stripped of multiple electrons, have been used to create strong electric field environments for experiments. As the atomic number increases and the shielding effect [10] is suppressed by the stripping off of many electrons, the remaining electrons in a polyvalent ion feel the electric field more strongly. Therefore, the production of polyvalent heavy ions using large accelerators is an active area of research. However, the influence of the size of the nucleus cannot be ignored, even with polyvalent heavy ions with large atomic numbers and those that are as small as about 1 femtometer (1/1,000 trillionth of a meter). The fact that this effect is not fully understood greatly compromises the accuracy of QED studies aimed at comparing experimental results to theory.
Methods and Findings
A characteristic of muonic atoms is that the orbital radius of the negative muons bound to the nucleus is about 1/200th that of a bound electron, putting the muons extremely close to the nucleus. As a result, the electric field felt by the negative muons can be about 40,000 times stronger than the electric field felt by bound electrons at the same quantum level in polyvalent ions, creating an extremely large QED effect. In addition, when negative muons, which occupy a quantum level with high angular momentum and little overlap with the nucleus, are used in measurements, it is possible to suppress the influence of the size of the nucleus to such an extent that it can be safely ignored.
Precise measurements of the energy that muonic X-rays emit during the transition (deexcitation) of muonic atoms from a certain energy level to a lower one creates an environment in which QED can be studied under a stronger electric field than possible with polyvalent heavy ions (Fig. 1).
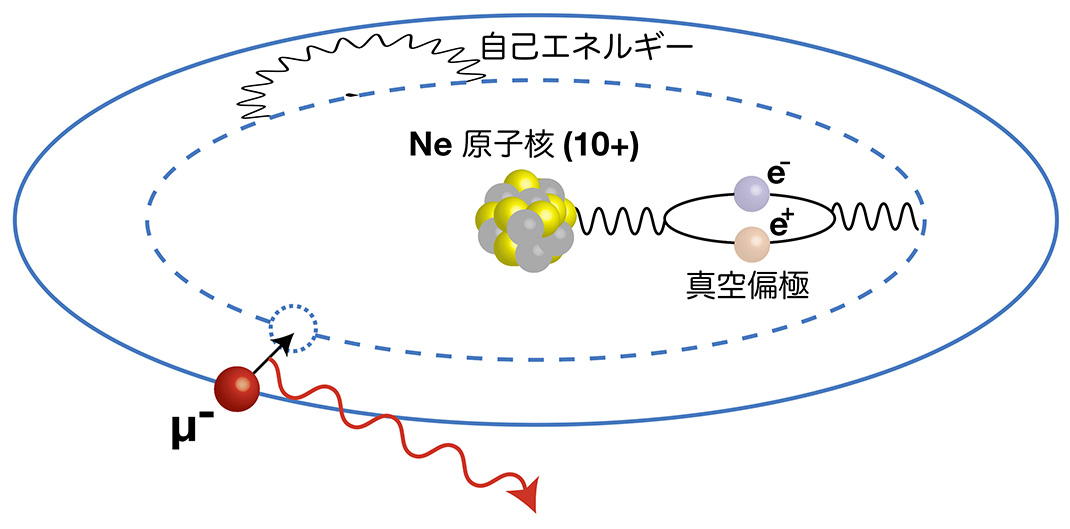
Figure 1. Conceptual diagram of muonic atoms and quantum electrodynamics (QED) effects
To counter this, the researchers increased the amount of muonic atoms that were generated as much as possible through experiments at the Muon Science Experimental facility (MUSE) D line, which is part of J-PARC's Materials and Life Science Experimental Facility (MLF) in Tokaimura, Ibaraki Prefecture, and is capable of generating the world's strongest low-speed muon beam. In addition, to determine energy levels accurately enough for low-intensity muonic X-rays, they used a highly sensitive, high-resolution X-ray detector called a superconducting Transition-edge microcalorimeter (TES).
Using atoms of the noble gas neon (Ne: atomic number 10), as the target, they achieved an energy resolution 10 times greater than conventional semiconductor detectors under rarefied conditions of 0.1 atm (full width at half maximum [11] 5.2 eV) to successfully measure the muonic X-rays emitted by the muonic Ne atoms (Fig. 2). The peaks shown in Figure 2 represent overlapping muonic X-rays from 6 main types of transitions. The muonic X-ray energy was determined with an extremely high accuracy of 0.002% by analyzing each of their contributions.
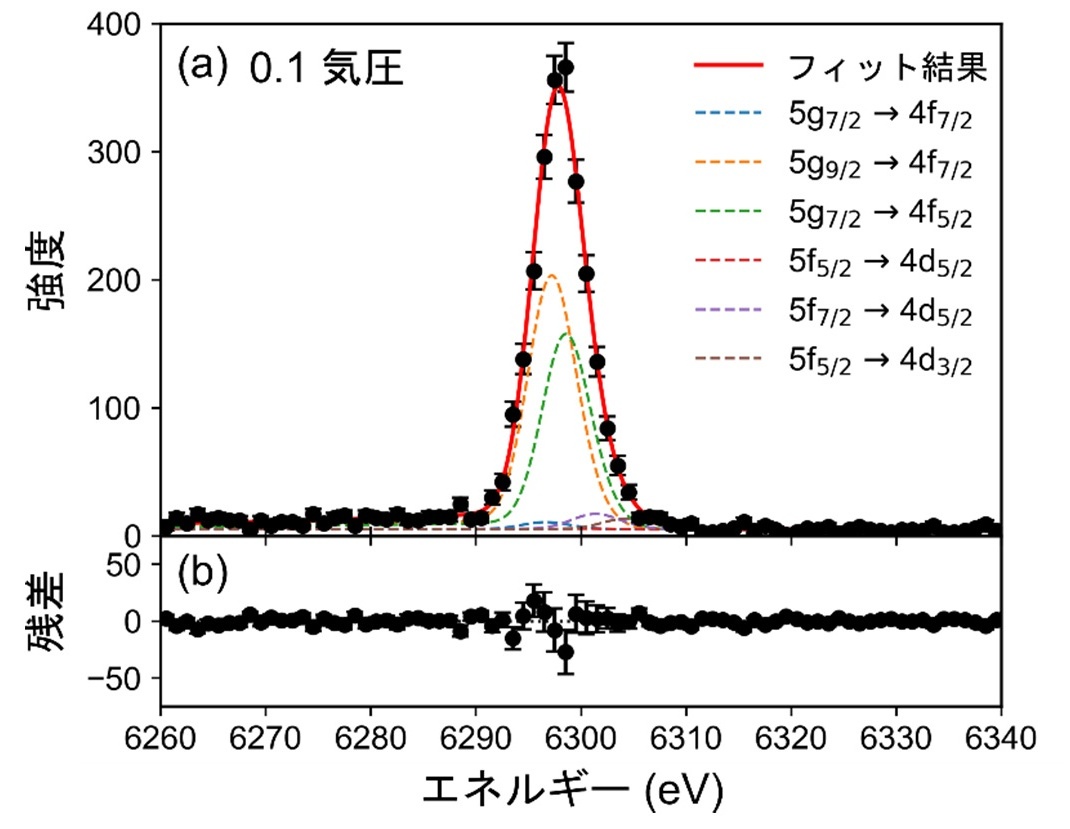
Figure 2. Spectrum of muonic X-rays emitted from muonic Ne atoms
(b) This shows the residuals (differences between predicted and actual values) from fitting . The small size of the residuals shows that fitting was highly accurate.
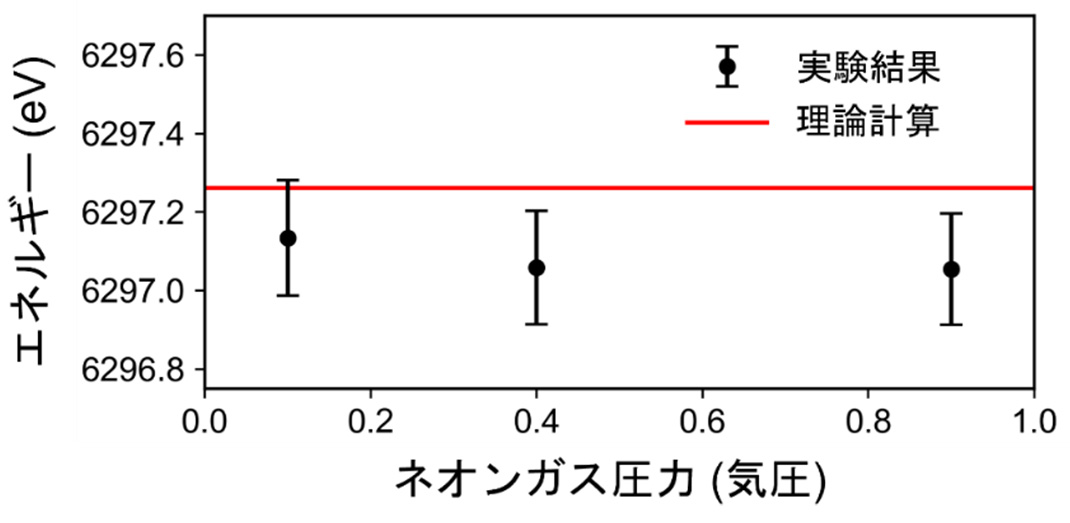
Figure 3. Comparison of the muonic X-ray energy under different neon gas pressures with the latest theoretical calculations
Future prospects
In the world of ultra-strong electric fields that exceed the threshold called the Schwinger limit [12], theory predicts that higher-order QED effects, which have not yet been observed, will be noticeable. This challenge awaits. With polyvalent ions, even when using uranium (92U), which has a very high atomic number, the Schwinger limit cannot be exceeded, but with muonic atoms, even argon (18Ar), which has a low atomic number, can be used to create a strong electric field that exceeds this limit. This demonstration of the research group's experimental method with muonic atoms is expected to lead to major strides in research on verifying QED under strong electric fields.
Recently, muonic atoms have also attracted attention as a tool for nondestructive element analysis. Applying the method of precisely measuring muonic X-ray energy established in this study in elemental analysis could help develop new fields of research such as in traditionally difficult isotope analyses, as well as in the analysis of the chemical states of elements.
Article information
- Title
Proof-of-Principle Experiment for Testing Strong-Field Quantum Electrodynamics with Exotic Atoms: High Precision X-ray Spectroscopy of Muonic Neon
- Authors
Takuma Okumura, Toshiyuki Azuma, Douglas A. Bennett, I-Huan Chiu, William B. Doriese, Malcolm S. Durkin, Joseph W. Fowler, Johnathon D. Gard, Tadashi Hashimoto, Ryota Hayakawa, Gene C. Hilton, Yuto Ichinohe, Paul Indelicato, Tadaaki Isobe, Sohtaro Kanda, Miho Katsuragawa, Naritoshi Kawamura, Yasushi Kino, Kairi Mine, Yasuhiro Miyake, Kelsey M. Morgan, Kazuhiko Ninomiya, Hirofumi Noda, Galen C. O'Neil, Shinji Okada, Kenichi Okutsu, Nancy Paul, Carl D. Reintsema, Dan R. Schmidt, Koichiro Shimomura, Patrick Strasser, Hirotaka Suda, Daniel S. Swetz, Tadayuki Takahashi, Shinichiro Takeda, Satoshi Takeshita, Motonobu Tampo, Hideyuki Tatsuno, Yasuhiro Ueno, Joel N. Ullom, Shin Watanabe, and Shinya Yamada
- Journal
Physical Review Letters
- DOI
10.1103/PhysRevLett.130.173001
Footnotes
A microcalorimeter is a detector used to measure the X-ray energy generated from the rise in temperature when a substance absorbs X-rays. A transition-edge microcalorimeter is composed of a thin film superconductor near the critical temperature, and changes in electrical resistance greatly depend on the temperature change of the thin film. Therefore, the changes in the current can be measured to determine the energy of the absorbed X-rays. TES has extremely high energy resolution and has recently been used in a wide range of scientific applications from astrophysics to materials science.
[2] Negative muons, muonic atoms
Muons are elementary particles that are second-generation charged leptons in the Standard Model. There are positively and negatively charged muons, both of which have a spin of 1/2 and a mean lifetime of 2.2 microseconds (1 microsecond is 1 millionth of a second). Negative muons collapse into electrons, muon neutrinos, and electron antineutrinos by the weak interaction. Because negative muons are about 200 times heavier than electrons and are also negatively charged, they also bind to positively charged nuclei and behave like "heavy electrons." Atoms composed of negative muons and nuclei are called muonic atoms. Muonic atoms eventually decay at the end of the life of the negative muon or when the negative muon is captured by the nucleus.
[3] Muonic X-rays
When a negative muon bound to a nucleus transitions from a high-energy orbit to a low-energy one, the excess energy is emitted as X-rays. These X-rays have an inherent energy from the atom and are called muonic X-rays.
[4] Quantum electrodynamics
Field theory states that the electromagnetic interaction works through the exchange of photons between charged particles. This has successfully explained various phenomena that could not be understood by relativistic quantum mechanics.
[5] Exotic atoms
An atom formed by replacing its nucleus or electrons with another charged particle (positron, muon, antiproton, pion, etc.) is called an exotic atom. The combinations of the charges and masses of the particles that make up exotic atoms are very different from those of electrons and nuclei, making them behave in interesting ways that differ from ordinary atoms.
[6] Nondestructive elemental analysis using muonic atoms
A method of measuring the electronic X-rays emitted when a sample is irradiated with high-energy X-rays or electron beams has been widely used in non-destructive element analysis of unknown samples. However, the electronic X-rays emitted by lighter elements such as carbon and hydrogen have low energy, which makes accurate elemental analysis difficult. Recently, a method of nondestructive elemental analysis that uses the muonic X-rays emitted when a sample material is irradiated with a negative muon beam has been established. Because muonic X-rays generally have higher energy than electronic X-rays, this method is extremely sensitive to light elements. Elemental analysis using muons has been used with samples brought back from the asteroid Ryugu, demonstrating the usefulness of this technique.
[7] Japan Proton Accelerator Complex (J-PARC)
J-PARC is an accelerator facility in Tokai, Ibaraki Prefecture, where cutting-edge research using one of the highest intensity proton beams in the world is conducted in fields including elementary particles, atomic physics, materials, and life sciences. The muon experimental facility (MUSE) in J-PARC's Materials and Life Science Experimental Facility (MLF) is capable of generating the world's most intense low-velocity muon beam.
[8] Vacuum polarization
Quantum electromagnetic effects on muonic atomic energy are mainly classified into two types: vacuum polarization and self-energy. According to quantum electrodynamics, a virtual particle is one that exists momentarily as an intermediate state in interparticle interactions. In the photons responsible for electromagnetic interactions, virtual electron-positron pairs are constantly forming and disappearing. The energy change associated with the formation and disappearance of virtual electron-positron pairs is called vacuum polarization. In addition, bound muons in muonic atoms are constantly absorbing and emitting virtual photons, and the energy change caused by this process is called self-energy. It is known that the effect of vacuum polarization is particularly large in the quantum electromagnetic effect on muonic atoms. For muonic Ne atoms, the effect of vacuum polarization is more than 1,000 times greater than self-energy.
[9] Transition energy
In quantum mechanics, muons and electrons bound to a nucleus must move in discrete orbits, and their energies are discretized (quantized) accordingly. The excess energy generated when a particle transitions from a high-energy orbit to a low-energy orbit is called transition energy. In many cases, transition energy is emitted as electromagnetic waves (light or X-rays).
[10] Shielding effect
This occurs in atoms with multiple electrons when the Coulomb force between the electrons and the nucleus appears to decrease due to the charge of the remaining electrons.
[11] Full width at half maximum
This describes the width when a value is half of a certain observation value. With detectors, the smaller the full width at half maximum, the higher the resolution of the observations, which allows for precise measurements of peak values.
[12] Schwinger limit
According to quantum electrodynamics, in ultra-strong electric fields of intensity greater than 1.32×1018 V/m, nonlinear effects appear in the electromagnetic field. This extreme electric field is called the Schwinger limit after physicist Julian Schwinger.
International research group
RIKEN and Chubu University in particular played leading roles in supervising and leading the study overall, with NIST contributing greatly to developing the detector. The KEK Institute of Materials Structure Science played a major role in the development and operation of the low-speed negative muon beams and in installing the experimental equipment, while the Kavli Institute for the Physics and Mathematics of the Universe, Osaka University, JAEA, Tokyo Metropolitan University, Rikkyo University, Kastler-Brossel Laboratory, Tohoku University, and JAXA were drivers of the development of the experimental equipment and measurement systems, and in acquiring and analyzing the experimental data.
- RIKEN
Atomic, Molecular, and Optical Physics Laboratory, RIKEN Cluster for Pioneer Research
Postdoctoral researcher (during the study): Takuma Okumura (now an assistant professor at the department of chemistry in the Graduate School of Science at Tokyo Metropolitan University)
Senior researcher: Toshiyuki Azuma
Advanced research fellow (during the study): Yasuhiro Ueno
Radioactive Isotope Physics Laboratory, RIKEN Nishina Center for Accelerator-Based Science
Senior researcher: Tadaaki Isobe
- U.S. National Institute of Standards and Technology (NIST)
Researcher: Douglas A. Bennett
Researcher: William B. Doriese
Researcher: Malcolm S. Durkin
Researcher: Joseph W. Fowler
Researcher: Johnathon D. Gard
Researcher: Gene C. Hilton
Researcher: Kelsey M. Morgan (researcher with the Department of Physics, College of Engineering and Applied Science, University of Colorado, Boulder)
Researcher: Galen C. O'Neil
Researcher: Carl D. Reintsema
Researcher: Dan R. Schmidt
Researcher: Daniel S. Swetz
Researcher: Joel N. Ullom
- Department of Chemistry, Graduate School of Science, Osaka University
Assistant professor (during the study): Kazuhiko Ninomiya (now associate professor at the Radioisotope Research Center, Institute for Radiation Science, Osaka University)
Project researcher: I-Huan Chiu (now a postdoctoral fellow at the JAEA Materials Science Research Center) Department of Earth and Space Science
Assistant professor: Hirofumi Noda
- Japan Atomic Energy Agency (JAEA), Advanced Science Research Center
Deputy research director: Tadashi Hashimoto
- Department of Physics, Graduate School of Science, Tokyo Metropolitan University
Visiting researcher: Hideyuki Tatsuno
Graduate student (during the study): Ryota Hayakawa (now researcher at the International Center for Quantum-field Measurement Systems for Studies of the Universe and Particles, High Energy Accelerator Research Organization)
Graduate student (during the study): Hirotaka Suda
- Department of Physics, Rikkyo University College of Science
Associate professor: Shinya Yamada
Assistant Professor (during the study) Yuto Ichinohe (now researcher on the Data System Team, Nishina Center for Accelerator-Based Science, RIKEN)
- Kastler-Brossel Laboratory (France)
Professor: Paul Indelicato
Researcher: Nancy Paul
- Institute of Materials Structure Science, High Energy Accelerator Research Organization (KEK)
Professor: Koichiro Shimomura
Distinguished professor (during the study, now a researcher): Yasuhiro Miyake
Distinguished associate professor: Naritoshi Kawamura
Research institute lecturer (during the study, now a lecturer): Patrick Strasser
Assistant professor: Sotaro Kanda
Assistant professor (during the study, now a research institute lecturer): Soshi Takeshita
Researcher: Tanpo Motonobu
- Kavli Institute for the Physics and Mathematics of the Universe (Kavli IPMU), University of Tokyo Institute for Advanced Study
Professor: Tadayuki Takahashi
Specially appointed assistant professor: Shinichiro Takeda
Project researcher: Miho Katsuragawa
Graduate student (during the study) Kairi Mine
- Graduate School of Science, Tohoku University
Associate professor (during the study, now a professor): Yasushi Kino
Assistant professor (during the study): Kenichi Okutsu (now assistant professor, Department of Chemistry, Faculty of Science, Gakushuin University)
- Chubu University, Faculty of Engineering, Department of Creative Science and Engineering Experimental Education
Associate professor (during the study): Shinji Okada (now professor, Department of Mathematical and Physical Sciences, Faculty of Science and Technology)
- Department of Space Astronomy and Astrophysics, Institute of Space and Astronautical Science (ISAS), Japan Aerospace Exploration Agency (JAXA)
Assistant professor (during the study, now associate professor): Shin Watanabe
Research funding
Other News
-
Apr 09, 2024
PRESS RELEASE