Jun 22, 2023PRESS RELEASE
Image Reconstruction Technique to Enhance the Clarity of High Spatial Resolution Space X-ray Images
~ Revealing fine structures in Cassiopeia A Supernova Remnant ~
Keyword:RESEARCH
OBJECTIVE.
A research team from Yamada Laboratory at the Department of Physics, Graduate School of Science, Rikkyo University, consisting of Yusuke Sakai (a graduate student), Shinya Yamada (an associate professor), Toshiki Sato (an assistant professor, currently a lecturer at Meiji University), Ryota Hayakawa (a researcher, currently at KEK), Ryota Higurashi (formerly a graduate student at Rikkyo University), and Nao Kominoto (a graduate student), successfully developed a novel image reconstruction technique, or deconvolution method, by making the best use of the world's highest angular resolution of the Chandra X-ray Observatory. Using this method, we have successfully enhanced the clarity of the X-ray image of the Cassiopeia A supernova remnant. Figure 1 (left) shows an observation image from Chandra, while Figure 1 (right) presents the results after applying our technique. By applying this new image analysis method, it becomes possible to accurately estimate the spatial extent of X-rays produced by high-energy cosmic phenomena, which can lead to improved measurement accuracy and the discovery of unknown structures. In addition, this method can be applied not only to Chandra but also to other satellites and data, opening up various possibilities for development in astronomy, such as comparative studies with radio and visible light observations. This research achievement will be published online in "The Astrophysical Journal" on June 22, 2023 at 22:00 JST (June 22, 2023 at 9:00 ET).
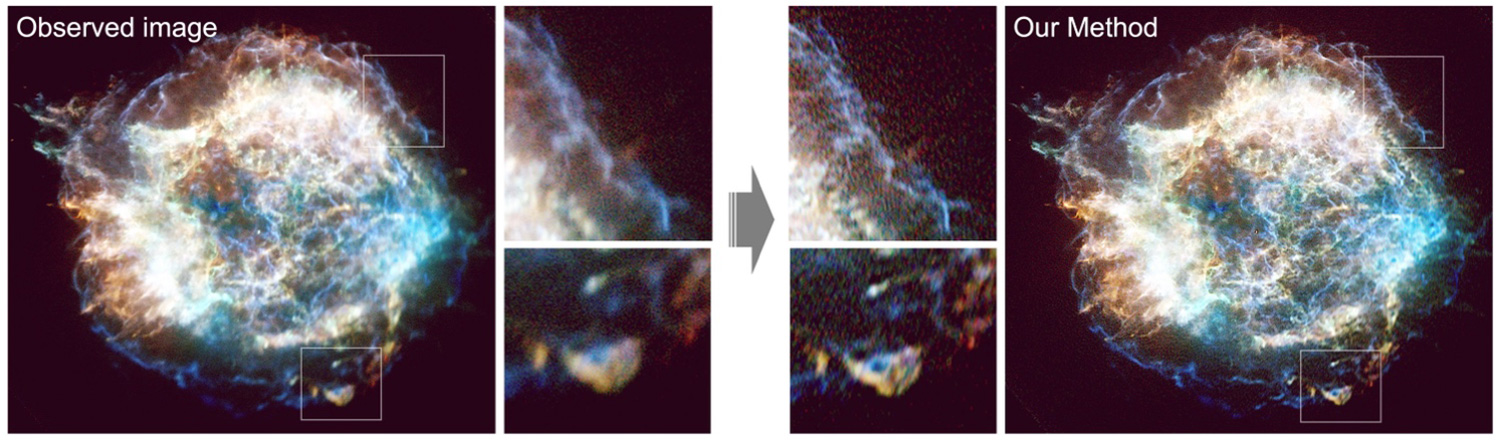
Figure 1: (Left) Observed image of the Cassiopeia A supernova remnant by the Chandra X-ray satellite. The colors correspond to the energy ranges of X-rays: red: 0.5-1.2 keV, green: 1.2-2.0 keV, blue: 2.0-7.0 keV. (Right) The result obtained by applying our method to the left image, resulting in improved clarity across the entire image. To view a high-resolution version, please click here.
Background of Research
The Gap Between Actual Observational Images and Real Celestial Objects
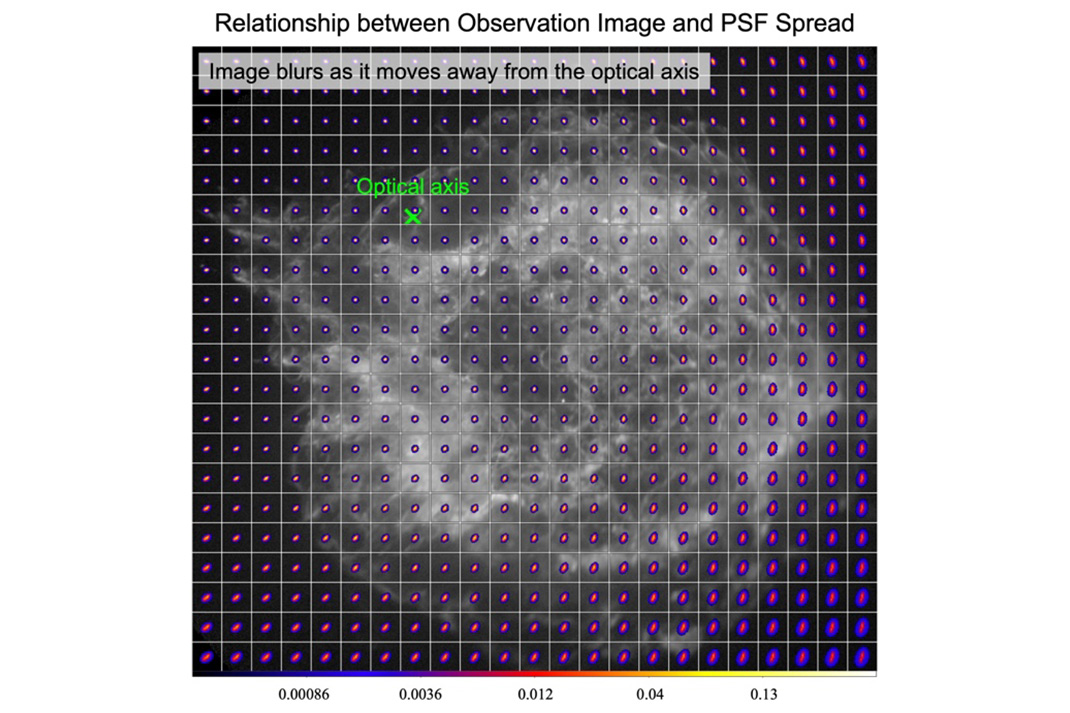
Figure 2: (Background) Monochromatic image of Cassiopeia A (Obs. ID=4636) observed by the Chandra in 2004. (Color image) Display of the simulated PSF for the monochromatic energy (2.3 keV), shown at regular intervals. The color represents the probability distribution of the PSF.
Among these X-ray satellites, Chandra, which has been operational since its launch in 1999 and continues to operate as of 2023, has the highest spatial resolution (0.5 arcseconds) of any X-ray satellite. Its exceptional capabilities have provided insights into various high-energy physical phenomena, including the time evolution of supernova remnants. However, the focusing device (X-ray telescope) that directly affects X-ray vision becomes less efficient at accurately focusing the image as we move away from the optical axis. The deviation of the image from the ideal point image is known as the point-spread function (PSF). Figure 2 shows the shape of the PSF at different locations based on simulated measurements using the observed image of the Cassiopeia A supernova remnant from Chandra in 2004 as the background. As the distance from the optical axis increases, the focusing power decreases and the PSF spreads wider. In other words, the observed image is not an accurate representation of the true celestial object, but rather a result of viewing it through lenses with varying focusing powers at different locations.
Method for correcting the effects of varying focusing power due to the direction of light incidence
Research results
Development of a deconvolution method applicable to the entire range of Chandra observations
Application to the Cassiopeia A supernova remnant
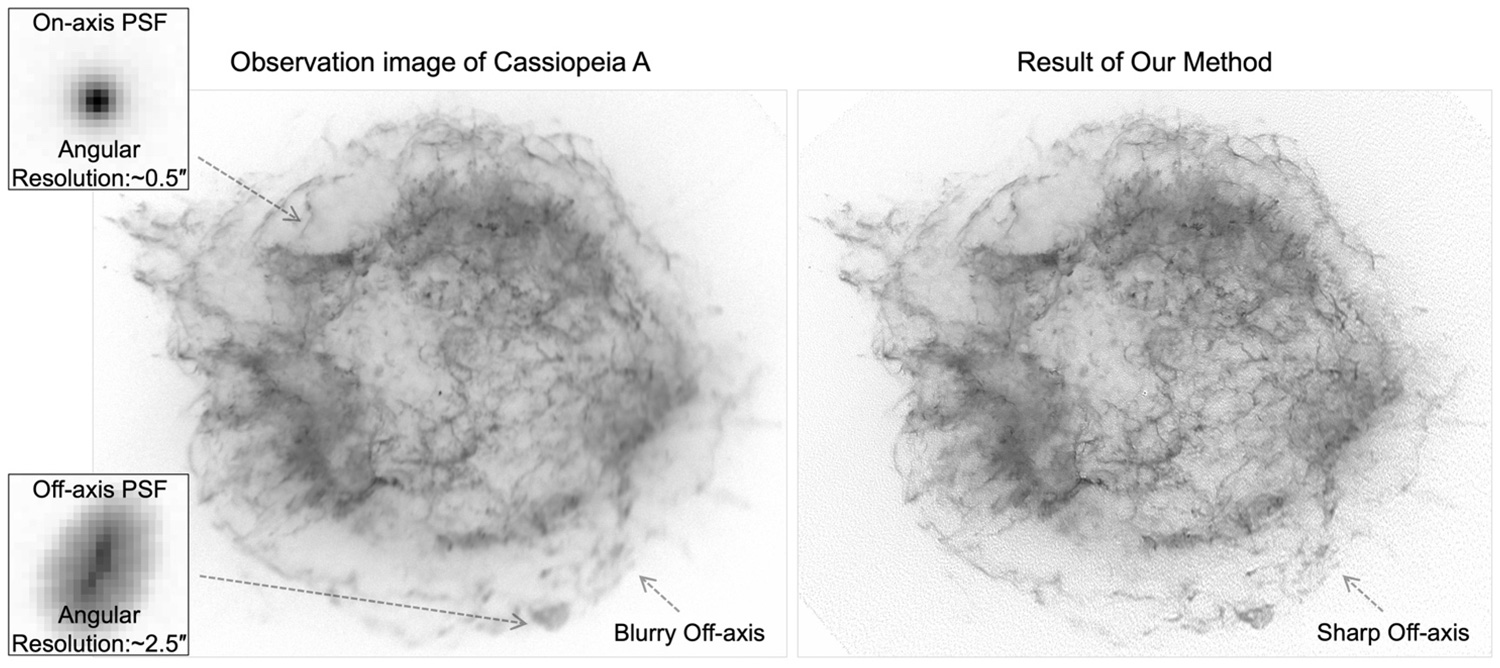
Figure 3: (Left) Observation image (0.5-7.0 keV, Obs. ID=4636, 4637, 4639, 5319). (Right) Result of 200 iterations of our position-dependent RL method using the PSF for each position shown in Figure 2, applied to the left image.