Mar 29, 2022PRESS RELEASE
High intensity accelerator × ultra-high precision "thermometer" to explore the power to create atomic nuclei
- Dramatically improved measurement accuracy of X-rays from exotic atoms -
Keyword:RESEARCH
OBJECTIVE.
An international research group succeeded in dramatically improving the accuracy of measurements of how the strong interaction affects negatively charged antikaons (K- kaons) Using the world's most intense kaon*2 beam provided by the Japan Proton Accelerator Research Complex (J-PARC*1) and an ultra-high-precision thermometer.
The team included Associate Professor Shinya Yamada and Assistant Professor Yuto Ichinohe of Rikkyo University; Associate Professor Sunao Hashimoto of the Research Group for Nuclear and Hadron Physics at the Advanced Science Research Center of the Japan Atomic Energy Agency; and Associate Professor Shinji Okada of the Center for Muon Science and Technology at the Engineering Science Laboratory of Chubu University.
Main points of the article
- In X-ray measurements, there is generally a trade-off between efficiency and resolution. Among the available methods, superconducting transition edge microcalorimeters, which use the most accurate thermometers in the world, have attracted attention recently for being X-ray detectors with high levels of both efficiency and resolution.
- For the first time ever, this research group operated a superconducting transition-edge microcalorimeter within the harsh radiation environment of the high-intensity beam at J-PARC. These measurements of the minute amounts of X-rays emitted from a type of exotic atom called kaonic atoms were 10 times more accurate than conventional methods. Kaonic atoms contain negatively charged antikaons (K- kaons) instead of electrons.
- The "strong interaction," one of the four fundamental forces of nature, also acts on K- kaons, and the attributes of this force affect the X-ray energy of the kaonic atoms. The strong interaction is the force that binds protons and neutrons together to form nuclei, and is also the source of nuclear energy. The team's findings provide important basic data for quantitatively exploring this phenomenon.
- This X-ray measurement technique is expected to lead to breakthroughs not only in the study of the strong interaction but also in X-ray measurements under different radiation environments, such as in accelerator beam experiments and astronomical observations.
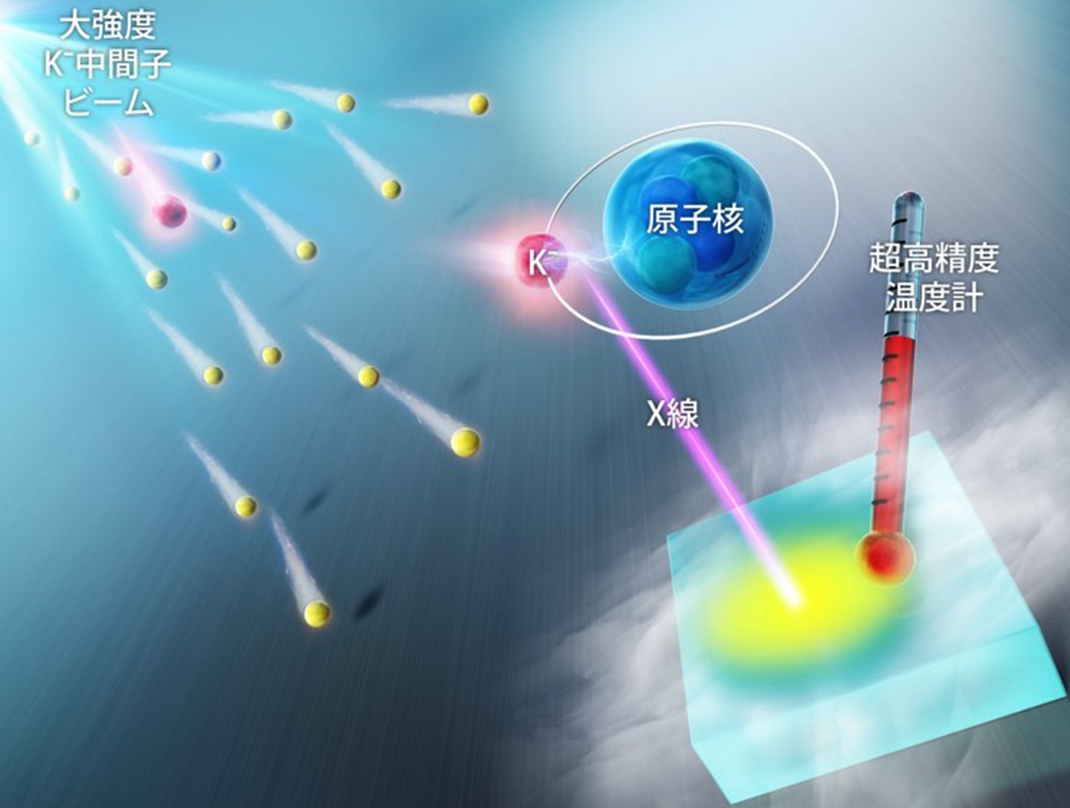
Figure 1: A K- kaon (red particles in the figure) beam contains many other particles (yellow particles in the figure), resulting in an extremely large number of charged particles flying around and a high level of radiation intensity. In this study, the energy of X-rays emitted from kaonic atoms was precisely measured under this kind of harsh radiation environment using an X-ray detector with an ultra-high-precision thermometer that operates at very low temperatures.
It has been pointed out that once K- kaons enter the nucleus, they may strongly attract multiple protons via the strong interaction, which forms a nucleus with higher than normal density. Thus, these particles have attracted attention recently as the key to producing high-density nuclear matter that does not exist on Earth. One of the most promising methods for investigating how the strong interaction acts on K- kaons is to produce kaonic atoms*4, which are a type of exotic atom that have incorporated kaons supplied by an accelerator, and measure their X-ray energy. However, the accuracy of these measurements in previous experiments has been low due to the limited intensity of K- kaon beams, which makes it difficult to obtain a large X-ray signal, and because the performance of the X-ray detector is degraded by the high levels of radiation in accelerator experiments.
This study included a superconducting transition-edge microcalorimeter, which is an X-ray detector that combines excellent energy resolution with high detection efficiency. This detector uses a highly sensitive thermometer made with cryogenic technology to convert X-ray energy into heat to achieve highly accurate measurements. Because the detector is sensitive to disturbances, it was thought it could be difficult to use it with accelerator beamlines that have a large number of particles flying around. However, the team established a method capable of fully demonstrating the detector's performance even in harsh radiation environments by optimizing the entire experimental setup and methods of data analysis. As a result, they successfully measured the X-ray energy emitted from kaonic atoms at 10 times the precision than previously achieved.
This provides important basic data on the strong interaction that acts on K- kaons. Accumulating more precise data using similar methods could help to explain the strong interaction, including in high-density regions that remain unexplored, and to better understand the origins of matter. In addition, the method established by this group is expected to lead to great leaps forward not only in the study of the strong interaction but also in X-ray measurements under different radiation environments, such as in muonic atom X-ray measurements and other accelerator beam experiments and for astronomical observations with X-ray satellites.
The findings were posted online on March 18 (U.S. time) prior to publication in "Physical Review Letters," an American physics journal.
Background and History
K- kaons are mesons that contain a particular type of quark called a strange quark, which is not found in protons or neutrons. Previous research has shown that the strong interaction between kaons and protons produces a very strong attraction. This is in contrast to the strong interaction between protons and neutrons, which is repulsive at short distances and prevents nuclei from collapsing. It is believed that in nuclei, K- kaons attract protons to form nuclei with higher-than-normal densities. In fact, the existence of kaon-bound nuclei*5, in which a K- kaon is bound to 2 protons by the strong interaction, has been reported. Normally, 2 protons alone cannot create a bound state, but including a K- kaon would likely create very interesting novel nuclei. Obtaining detailed measurements of the strong interaction acting on K- kaons is rapidly growing in importance for understanding such nuclei.
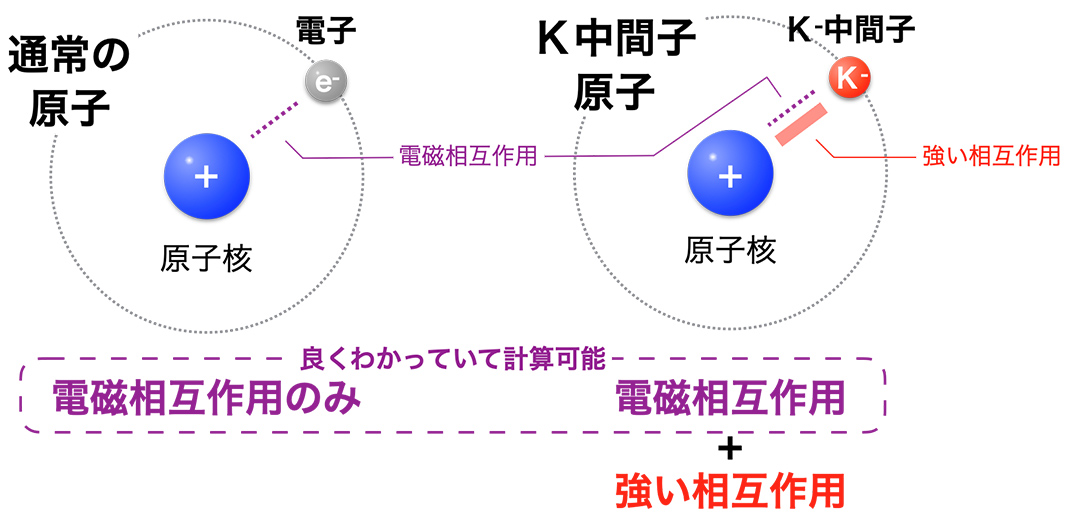
Figure 2: Schematic of kaonic atoms. Normal atoms are systems in which a positively charged nucleus and negatively charged electrons are bound by the electromagnetic interaction (left figure), while kaonic atoms are those in which K- kaons are bound instead of electrons, and the strong interaction acts in addition to the electromagnetic interaction between them and the nucleus (right figure). Because K- kaons are 1,000 times heavier than electrons, they are 1,000 times closer to the nucleus, and the strong interaction takes place over this short distance.
Kaonic atoms are produced by stopping an accelerator-generated K- kaon beam over a target material. However, the intensity of the beam is limited because of the large amount of energy needed to produce K- kaons, which have short lifetimes and decay quickly. Therefore, the number of kaonic atoms that can be produced is not very large. Further, because X-rays are emitted from kaonic atoms in all directions, the detector needs to have a large detection surface. Conventional experiments have used semiconductor detectors. However, recent theoretical developments have increased demand for detectors with higher levels of resolution that can produce dramatically more accurate measurements. Crystal spectrometers are often used as X-ray detectors because of their excellent energy resolution. However, their detection efficiency is so small that it can be difficult to detect enough X-rays, making these devices unsuitable for the group's experiment.
Research results
Superconducting transition-edge sensors (TES) utilize a phenomenon called "superconductivity," in which electrical resistance suddenly drops to zero when a specific material is cooled to a very low temperature. The very narrow temperature region just before the transition to superconductivity functions as an extremely sensitive thermometer because electrical resistance changes rapidly in response to temperature changes. To measure X-ray energy with this kind of high-precision thermometer, the energy is first converted into heat by causing the X-rays to react with a substance called an absorber. Measuring the resulting minute temperature increase with a TES thermometer can be used to determine X-ray energy at high levels of precision (Figure 3 left). This kind of detector is called a TES microcalorimeter (or TES detector). Compared to crystal spectrometers, which also have excellent energy resolution, a TES detector is superior in that its effective area can be expanded by using multiple elements to cover a wide energy range.
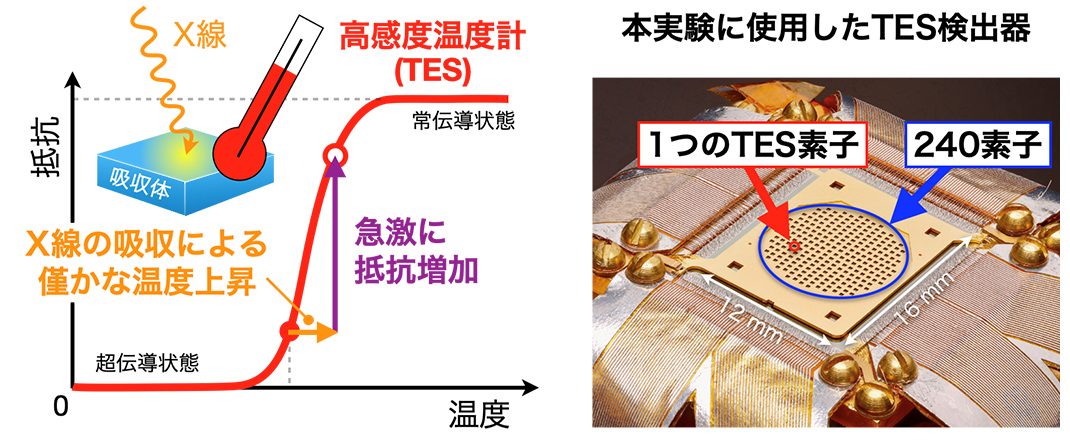
Figure 3: The figure on the left illustrates the principle of a TES detector. The slight temperature increase in the absorber caused by incident X-ray energy is measured at a high level of accuracy as a sudden increase in resistance of the TES thermometer. The photo on the right shows the TES detector used in this experiment. This is a 240-element device manufactured by the U.S. National Institute of Standards and Technology (NIST), which took part in the study, and is used by cooling it to about 0.1K.
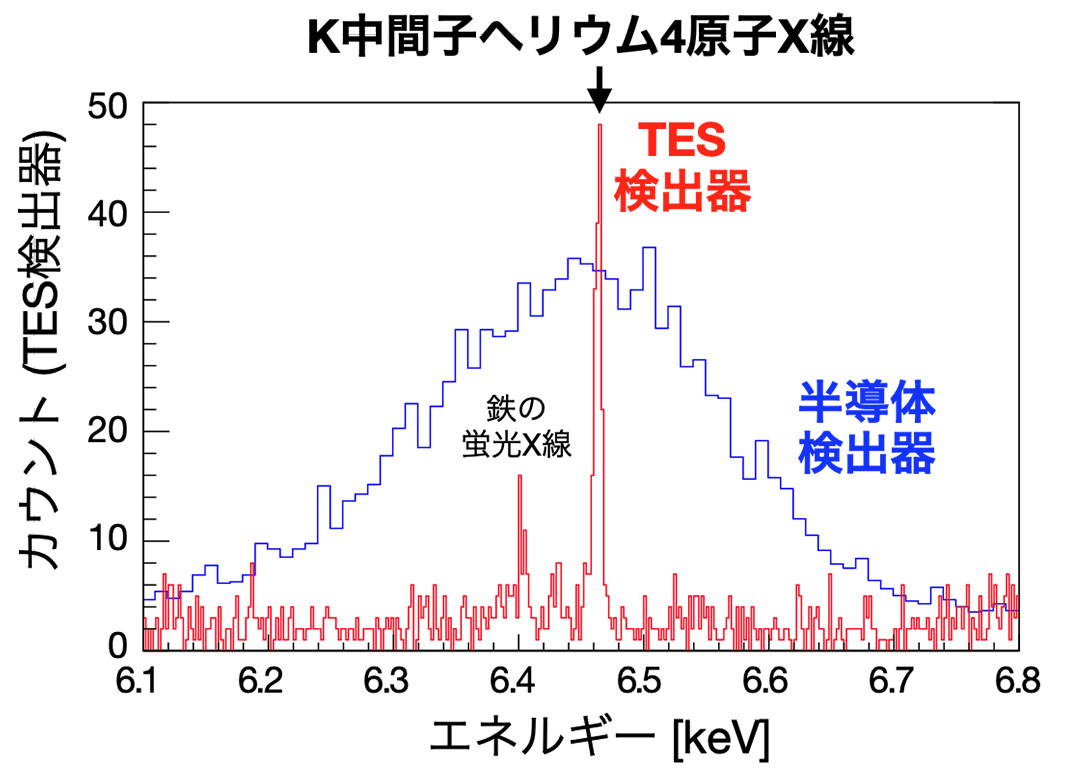
Figure 4: Energy spectra of X-rays (3d→2p transition) from 4 kaonic helium atoms measured in this study. The red line corresponds to the spectra measured with a TES detector and the blue to that from a conventional semiconductor detector. The TES detector provided overwhelmingly better resolution. This X-ray has a narrower energy distribution than peaks observed with conventional TES detectors. Moreover, with a semiconductor detector the peak is broader and more "blurry" due to the detector's performance. This figure also shows that contaminants (X-ray fluorescence of iron) that the semiconductor detector could not isolate can be measured as separate peaks using the TES detector.
Future prospects
This study was an international collaboration with the U.S. National Institute of Standards and Technology (NIST), a world leader in the development of TES detector technology, involving a multi-disciplinary team of researchers who specialize not only in nuclear and hadron physics, but also in atomic, molecular, and astro-physics, and are engaged in developing TES detectors to use on X-ray satellites. Their findings represent an important milestone for high-precision X-ray observation experiments performed in harsh radiation environments that have large amounts of charged particles flying around.
This experiment has prompted the team to embark on various other quantum beam experiments using TES detectors, such as for verifying fundamental physical laws through muonic atom X-ray measurements and earth and environmental research by X-ray absorption edge measurements at synchrotron radiation facilities. The team is currently developing TES detectors for a wider range of X-ray energies that can be used in X-ray spectroscopy experiments in a broader range of fields.
Article information
- Journal: Physical Review Letters
- Title: Measurements of Strong-Interaction Effects in Kaonic-Helium Isotopes at Sub-Electron Volt Precision with X-Ray Microcalorimeters
- Authors: T. Hashimoto, S. Aikawa, T. Akaishi, H. Asano, M. Bazzi, D. A. Bennett, M. Berger, D. Bosnar, A. D. Butt, C. Curceanu, W. B. Doriese, M. S. Durkin, Y. Ezoe, J. W. Fowler, H. Fujioka, J. D. Gard, C. Guaraldo, F. P. Gustafsson, C. Han, R. Hayakawa, R. S. Hayano, T. Hayashi, J. P. Hays-Wehle, G. C. Hilton, T. Hiraiwa, M. Hiromoto, Y. Ichinohe, M. Iio, Y. Iizawa, M. Iliescu, S. Ishimoto, Y. Ishisaki, K. Itahashi, M. Iwasaki, Y. Ma, T. Murakami, R. Nagatomi, T. Nishi, H. Noda, H. Noumi, K. Nunomura, G. C. O’Neil, T. Ohashi, H. Ohnishi, S. Okada, H. Outa, K. Piscicchia, C. D. Reintsema, Y. Sada, F. Sakuma, M. Sato, D. R. Schmidt, A. Scordo, M. Sekimoto, H. Shi, K. Shirotori, D. Sirghi, F. Sirghi, K. Suzuki, D. S. Swetz, A. Takamine, K. Tanida, H. Tatsuno, C. Trippl, J. Uhlig, J. N. Ullom, S. Yamada, T. Yamaga, T. Yamazaki, J. Zmeskal
- DOI:10.1103/PhysRevLett.128.112503
Roles of the participating organizations
Joint Research Group (principal investigators from each institution)
S. Okada, Associate Professor, College of Engineering, Chubu University
S, Yamada, Associate Professor, College of Science, Rikkyo University
Y. Ishisaki, Associate Professor, Graduate School of Science, Tokyo Metropolitan University
F. Sakuma, Senior Researcher, RIKEN Cluster for Pioneering Research
H. Noumi, Professor, Research Center for Nuclear Physics, Osaka University
H. Onishi, Professor, Research Center for Electron Photon Science, Tohoku University
M. Sato, Institutional Lecturer, High Energy Accelerator Research Organization (KEK) Accelerator Research Complex
Grant Information
- Japan Society for the Promotion of Science (JSPS) grants-in-aid for scientific research (24105003, 25105514, 26707014, 26220703, 15H05438, 15H00785, 16H02190, 16H17718, 17J07791, 18H01237, 18H05402, 18H05458, 20K14524, 20K20527)
- Mitsubishi Foundation natural science grant (grant No. 26145)
- Ministry of Education, Culture, Sports, Science and Technology; Leading Initiative for Excellent Young Researchers (LEADER)
- RIKEN research incentive fund
- EU STRONG-2020 project (Grant No. 824093)
- HRZZ project 8570.
Glossary
- *1: Japan Proton Accelerator Research Complex (J-PARC)
Overall name for a group of high-intensity proton accelerators and utilization facilities in Tokai-mura, Ibaraki Prefecture. Using various secondary particle beams produced by the world's most intense proton beam, cutting-edge academic research and industrial applications in materials and life sciences, nuclear and particle physics, and other fields are being conducted at the complex. At the complex's hadron experimental facility, high-energy proton beams are directed at a gold target to produce secondary particle beams such as kaons and π mesons for research on elementary particles and nuclear hadrons. J-PARC is the abbreviation for the Japan Proton Accelerator Research Complex.
- *2: Meson, kaon
A meson occurs when a quark and an antiquark are bound together by the strong interaction. Of the four types of kaons, negatively charged antikaons (K- kaons) are composed of a strange quark and an up antiquark.
- *3: Quark, anti-quark
Quarks are elementary particles and one of the smallest units of matter, and have 6 types with 3 generations. Up/down quarks are the first generation, the lightest, and the most stable. The second generation is charm/strange quarks and the third is top/bottom quarks. All quarks have corresponding antiparticles (particles with opposing positive or negative attributes, such as charge) called antiquarks.
- *4: Kaonic atoms
An ordinary atom has electrons revolving around its nucleus, but in these particles negatively charged antikaons (K- kaons) revolve around the atom instead of electrons. While K- kaons and nuclei are bound together by electrical forces the same as in ordinary atoms, the strong interaction is also acting between K- kaons and nuclei.
- *5: Kaon-bound nuclei
The existence of a state in which kaons and nuclei are bound by the strong interaction has been predicted theoretically. Recently, the observation of kaon-bound nuclei called "K-pp," in which a kaon is bound to 2 protons, was reported. In kaon-bound nuclei, the kaons are embedded inside the nucleus, and even if they are generated, they decay quickly from reacting with the nucleus.
- *6: Neutron star
Neutron stars are ultra-dense stars composed mainly of neutrons that are produced by supernova explosions. They are one of the final forms that a star can take. With a radius just over 10 km and mass that is 1-2 times that of our sun, the density of these stars reaches 1 billion tons per cm3. These stars have also been called "giant nuclei" floating in space, and the high-density nuclear material at their center is believed to denser than that of ordinary nuclei.