Nov 21, 2019PRESS RELEASE
Harmony of triple forces: Ionic bonding, hydrogen bonding, and halogen bonding
An innovative technique to make iodine more functional and use it as a catalyst: Researchers have developed a new method to synthesize optically active lactone useful for drug development.
Keyword:RESEARCH
OBJECTIVE.
In their joint research, Professor Masayoshi Yamanaka of Rikkyo University’s Department of Chemistry and Professor Takayoshi Arai of Chiba University’s Department of Chemistry succeeded in developing a catalyst that facilitates steric selectivity reactions in a single metal complex by combining the processes of ionic bonding, hydrogen bonding, and halogen bonding (See Figure 1) (Arai also heads Soft Molecular Activation Research Center and Chiba Iodine Research Innovation Center).
<Outlines of the Research>
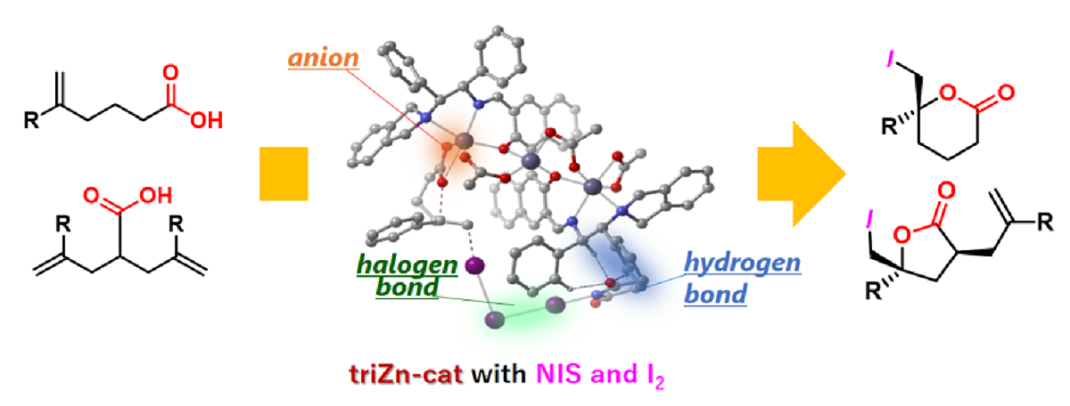
Figure 1 Cooperative activation using ionic bonding, hydrogen bonding, and halogen bonding in metal-catalyzed asymmetric halolactonization.
<Background and purposes>
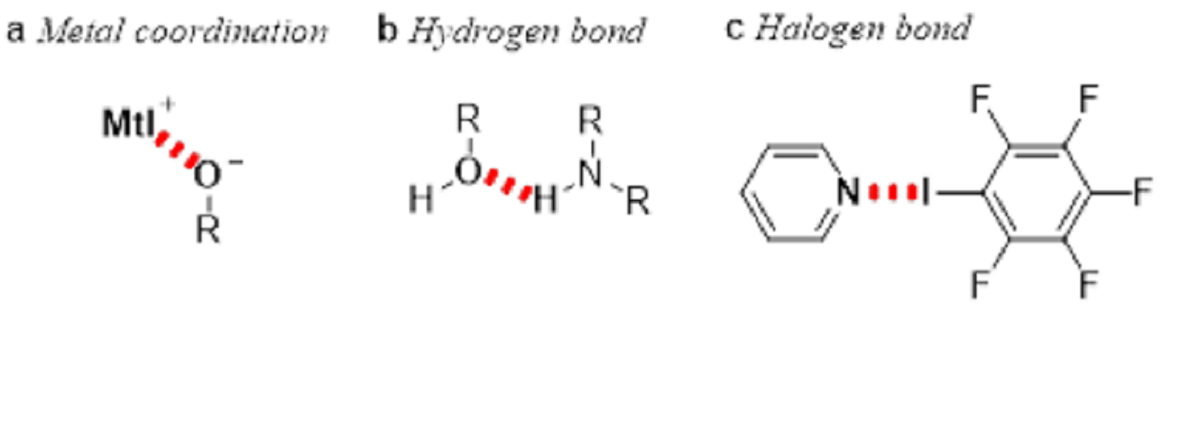
Figure 2 Three bonding powers fused in this study
For instance, metal catalysts are very attractive because they exhibit peculiar characteristics which are not seen in organic compounds alone (See Figure 2a). Furthermore, using complex catalysts with ligands that allow molecular recognition has made it possible to build complicated molecular frameworks. However, as this process uses environmentally harmful metal salts, it has become a major challenge to attain high levels of activation even by reducing the amount of catalysts as much as possible.
On the other hand, enzymes which play the roles of catalysts in our body perform efficient molecular recognition and activation using hydrogen bonding (See Figure 2b). This type of bonding is widely used in laboratory flask experiments to activate reaction substrates – particularly in the organic catalyst fields which do not use metals – as the most fundamental and effective catalyst mechanism.
To ensure state-of-the-art catalytic chemistry, incorporating the exceptional physiological function of the human body is essential. Fusing metal complex catalysis and organic catalysis is a subject that is actively being explored in research currently.
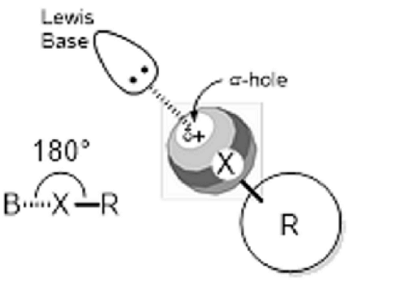
Figure 3: Halogen bonding
Accordingly, we consider halogen bonding – a new, third area of high potentiality. (See Figure 2c) Halogen bonding has attracted much attention as a new interaction with a clear direction which can have applications in catalytic chemistry and functional molecule creations. It was, however, difficult to achieve productive levels of chemical structural recognition, such as steric selectiveness, as halogen bonding is formed by a positive charge that exists behind an R-X bond in a molecular framework. (See Figure 3)
<Research results>
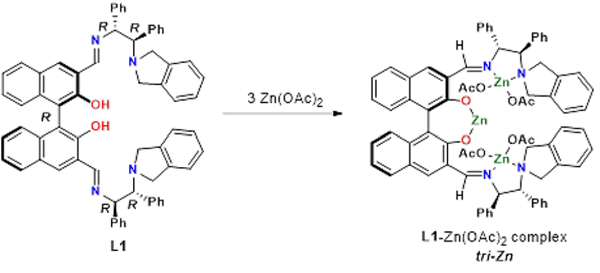
Figure 4: The zinc trinuclear complex catalyst (tri-Zn)
The zinc trinuclear complex catalyst (tri-Zn), which can easily be made from optically active Bis(aminoimino)biphenol ligands and zinc acetate, has three zinc atoms. (See Figure 4)
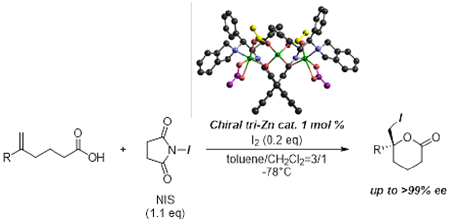
Figure 5: Tri-Zn-catalyzed asymmetric iodolactonization
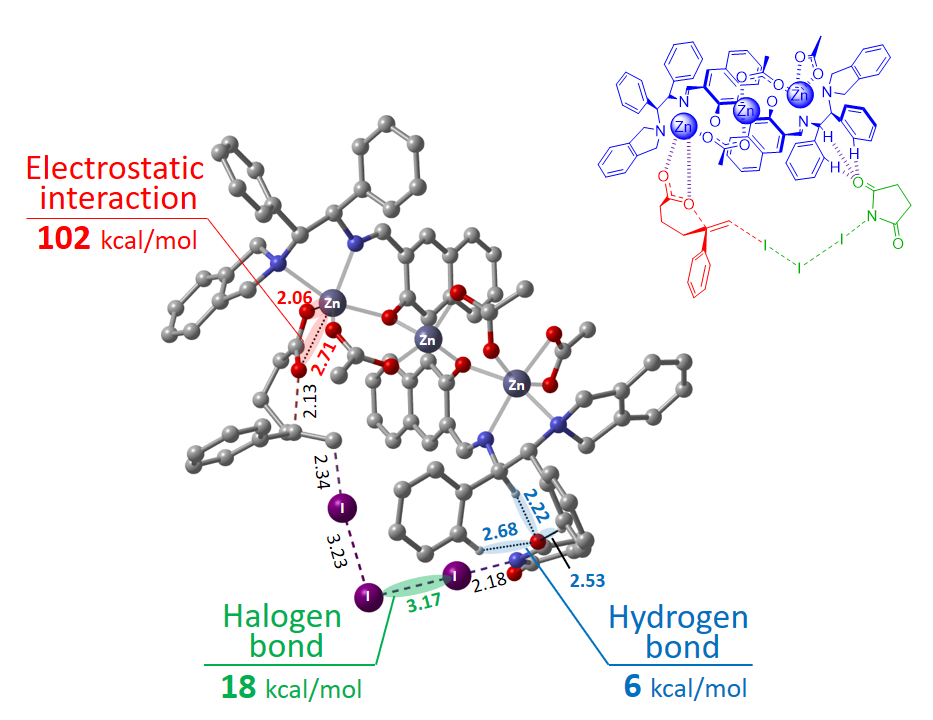
Figure 6: Reaction transition state in which ionic bonding, hydrogen bonding, and halogen bonding work in tandem.
DFT calculations were performed based on these findings to determine the transition state (See Figure 6). In the transition state, it was indicated that the substrate, zinc carboxylate, is activated by NIS-I2 reagent and that NIS is hydrogen-bounded with a ligand.
The catalyst is the world’s first catalyst to facilitate steric selective reactions by combining three types of bonding – ionic bonding, hydrogen bonding, and halogen bonding in a single metal complex (See Figure 6).
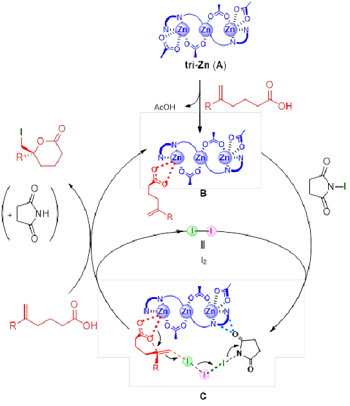
Figure 7: Catalytic cycle of asymmetric iodolactonization reactions with the use of tri-Zn complex
Figure 7 shows the researchers’ hypothesis on the catalytic cycle of asymmetric halolactonization reactions with the use of the tri-Zn complex. First, an acetate anion of the zinc trinuclear complex (A) exchanges with a substrate to generate zinc carboxylate (B). The olefin section of zinc carboxylate (B) is activated by the complex of NIS and iodine, reaching the aforementioned transition state (C) to help attain the desired iodolactonization. During the process, the olefin section is directly activated by I2 and not NIS. Therefore, if the reactions proceed as indicated in the curly arrows, I2 is regenerated. Therefore, 0.2 equivalent of I2 is sufficient to facilitate the reactions.
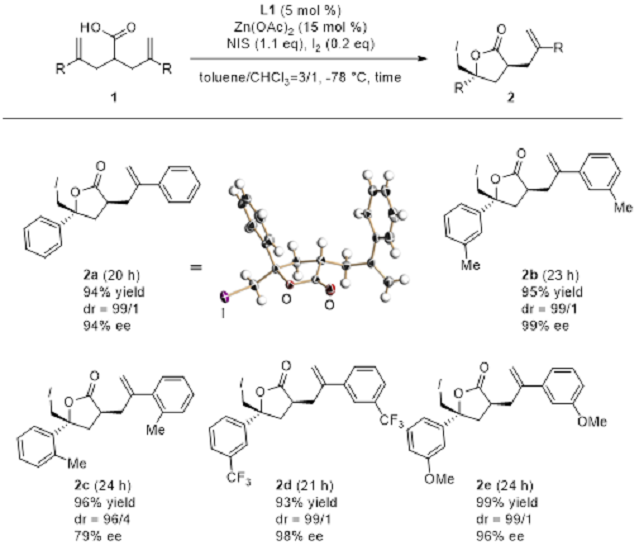
Figure 8: Asymmetric, 5-membered ring iodolactones by catalyst via antisymmetrization
Iodolectonization using a tri-Zn complex as catalyst on asymmetry substrate 1 will trigger reactions which progress with high diastereoselectivity, successfully resulting in the generation of 5-membered ring lactone 2 with a high asymmetric yield (See Figure 8).
The optically active 5-membered ring lactone 2 is highly usable for syntheses because it still contains an iodinated alkyl group and unreacted olefins in its molecules (See Figure 9).
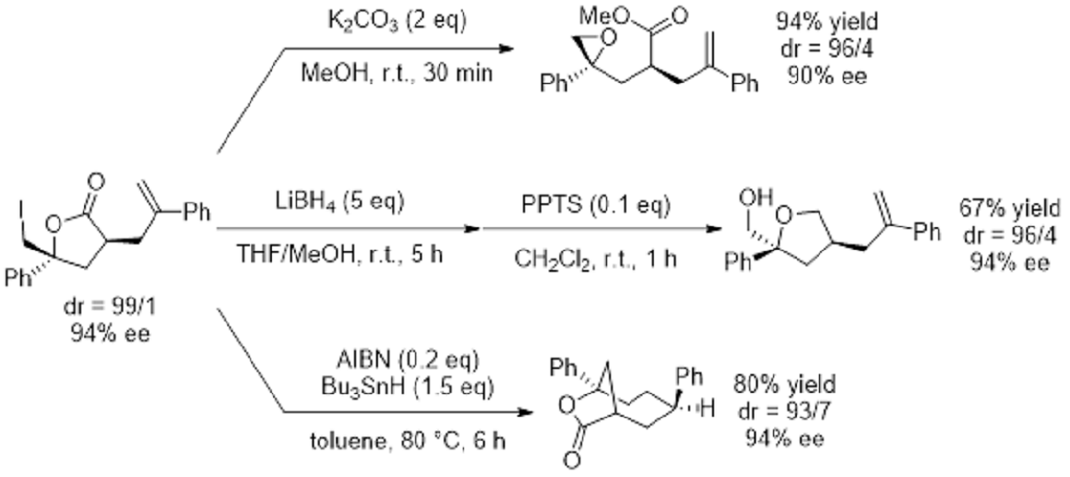
Figure 9: Usefulness of optical active 5-membered ring iodolactones via antisymmetrization
<Creativity and advancement>
<Social contribution and ripple effects>
The results of this study are published in iScience, an open access journal published by Cell-Press.
<Article>
2) Arai, T.; Kojima, T.; Watanabe, O.; Itoh, T.; Kanoh, H. ChemCatChem. 2015, 7, 3234.