Jul 26, 2021PRESS RELEASE
Describing the entire process of muonic atom formation using state-of-the-art superconducting detectors
- Femtosecond dynamics of negative muons, electrons, and atomic nuclei -
Keyword:INFORMATION
OBJECTIVE.
An international research group* used state-of-the-art X-ray detectors called transition-edge microcalorimeters (TES) [1] to precisely measure the energy spectra of electronic X-rays [3] emitted from muonic atoms [2] to comprehensively describe the dynamics of the muonic atom formation process. Their results are expected to open up new fields of research on the dynamics of exotic quantum few-body systems [4] composed of negative muons [2], electrons, and atomic nuclei. The research group included Specially Appointed Researcher Takuma Okumura, Chief Researcher Toshiyuki Azuma, and Collaborating Researcher Shinji Okada (now associate professor at Chubu University) of the RIKEN Cluster for Pioneering Research; Researcher Tadashi Hashimoto of the Japan Atomic Energy Agency; Associate Professor Shinya Yamada and Yuto Ichinohe of Rikkyo University; Visiting Researcher Hideyuki Tatsuno of Tokyo Metropolitan University; Professor Paul Indelicato of the Kastler-Brossel Laboratory; Associate Professor Xiao-Min Tong of Tsukuba University, Professor Tadayuki Takahashi of the University of Tokyo Kavli Institute for the Physics and Mathematics of the Universe; and Yasuhiro Miyake of the Institute of Materials Structure Science at the High Energy Accelerator Research Organization.
This study was selected as an Editors' Suggestion for publication in the online edition of the scientific journal "Physical Review Letters" on July 27.
1. Background
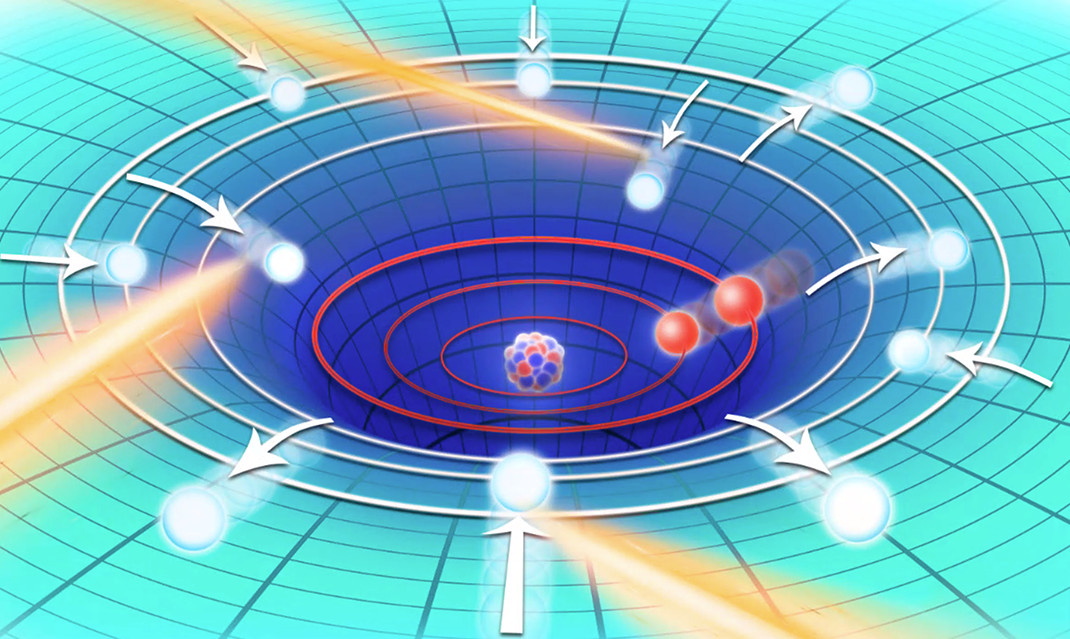
Figure 1. Dynamics of the muonic atom deexcitation process When negative muons (red spheres) are captured by an iron atom, a deexcitation cascade occurs and many bound electrons (white spheres) are emitted, which are then refilled by electrons from the surrounding area. Electronic K X-rays (orange rays) are emitted along with this.
The most important feature of muonic atoms is that the orbital radius of bound muons[6] is about 1/200 that of bound electrons, or extremely close to the nucleus. Previous research has examined the size and basic physics of muonic atoms in detail.
When a negative muon approaches an atom, it is initially captured at the excitation level[7] of the atom's orbit. It is then deexcited[7] by moving to levels below the excitation level while it strips many bound electrons from the atom. Further, the empty electron orbits in the atom created by the negative muon are refilled by bound electrons from the upper levels and electrons contained in the medium around the negative muon (Figure 1).
During the formation of muonic atoms, these processes involving negative muons and electrons occur in extremely rapid succession at a few dozen femtoseconds (fs, 1 fs = 1/1,000 trillionth of a second). Previously no experimental method had been capable of capturing the dynamics of muonic atom formation, and the whole picture of how negative muons move and how the number and arrangement of electrons change has remained unclear.
2. Methods and findings
However, differences in the energy of electronic X-rays need to be measured to a few dozen electronvolts (eV), but the energy resolution of the semiconductor detectors typically used to detect X-rays is only within a few hundred eV for every few thousand eV. Therefore, it has not been possible to capture detailed peaks of a few dozen eV, and due to this lack of resolution it has been difficult to accurately measure the shapes of electronic X-ray spectra.
Therefore, in this study the researchers used a new transition-edge microcalorimeter (TES), which is a high-resolution X-ray detector developed for observing cosmic X-rays, to measure the electronic X-rays emitted from the muonic iron atoms that formed by driving negative muons onto iron metal. The experiment was conducted at the Japan Proton Accelerator Complex (J-PARC) in Tokai, Ibaraki Prefecture, which is capable of generating the most intense ultra-slow muon beam in the world.
The experiment achieved more than an order of magnitude better energy resolution than previously (full width at half maximum (FWHM) [8] 5.2eV), and for the first time ever showed that the spectra of electronic Kα and Kβ X-rays [9] emitted from muonic iron atoms are both about 200 eV and have asymmetric shapes (Figure 2). They also discovered a type of electronic X-ray called hypersatellite (Khα) X-rays [9] that are emitted when there are 2 holes in the ground level of electrons (Figure 2).
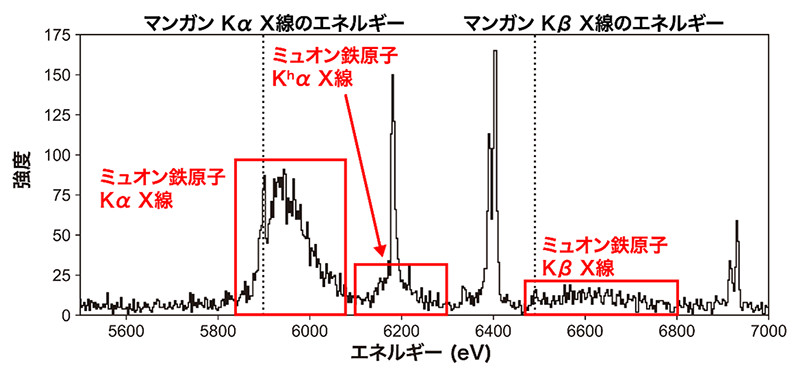
Figure 2. X-ray spectra of muonic iron atoms measured by transition-edge microcalorimeters
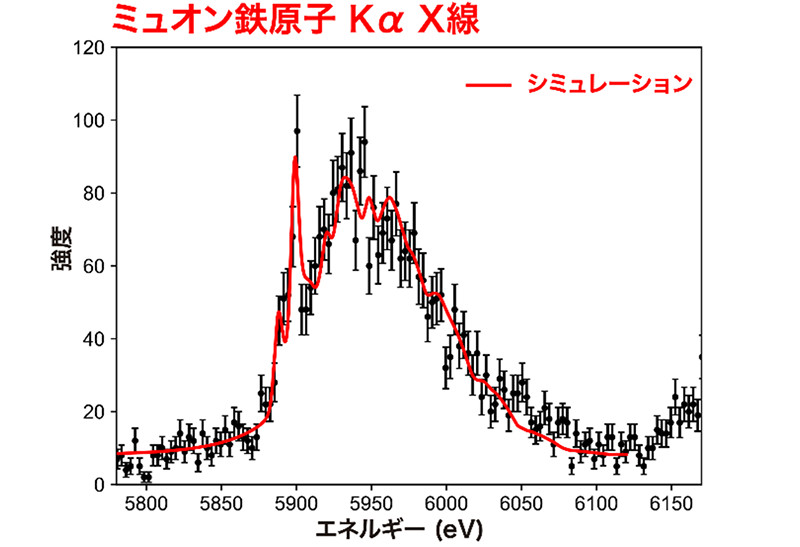
Figure 3. Comparison of simulations and experimental results of the muonic iron atom formation process
3. Future prospects
This method has a wide range of applications, and the electron filling rates of various substances obtained with it could be used like probes that are sensitive to the physical properties of different substances. Going forward, the research group aims to explore the possibility of applying these novel physical property probes not only to metals other than iron, but to insulators as well.
In addition, muonic atoms could serve as a stage for verifying the basic physics of extreme environments in that the presence, absence, or number of bound electrons in muonic atoms could support the accuracy of experiments. This study's description of how the process of muonic atom formation changes over time is expected to greatly improve the accuracy of experiments using muonic atoms.
4. Article information
- Title: De-excitation dynamics of muonic atoms revealed by high precision spectroscopy of electronic K x rays
- Authors: Takuma Okumura, Toshiyuki Azuma, Douglas A. Bennett, Pietro Caradonna, I-Huan Chiu, William B. Doriese, Malcolm S. Durkin, Joseph W. Fowler, Johnathon D. Gard, Tadashi Hashimoto, Ryota Hayakawa, Gene C. Hilton, Yuto Ichinohe, Paul Indelicato, TadaAki Isobe, Sohtaro Kanda, Daiji Kato, Miho Katsuragawa, Naritoshi Kawamura, Yasushi Kino, Kenya M. Kubo, Kairi Mine, Yasuhiro Miyake, Kelsey M. Morgan, Kazuhiko Ninomiya, Hirofumi Noda, Galen C. O'Neil, Shinji Okada, Kenichi Okutsu, Takahito Osawa, Nancy Paul, Carl D. Reintsema, Dan R. Schmidt, Kouichiro Shimomura, Patrick Strasser, Hirotaka Suda, Daniel S. Swetz, Tadayuki Takahashi, Shinichiro Takeda, Soshi Takeshita, Motonobu Tampo, Hideyuki Tatsuno, Xiao-Min Tong, Yasuhiro Ueno, Joel N. Ullom, Shin Watanabe, and Shinya Yamada
- Journal: Physical Review Letters (Editors’ Suggestion)
5. Supplemental explanations
A microcalorimeter is a detector used to measure the X-ray energy generated from the rise in temperature when a substance absorbs X-rays. A transition-edge microcalorimeter measures the temperature change from X-ray absorption using the steep change in electrical resistance near the phase transition between superconduction and normal conduction. By using superconducting transitions, even slight temperature changes can be detected as large changes in resistance, which enables high-resolution measurements of X-ray energy. TES is an abbreviation for Transition-Edge Sensor.
[2] Muonic atom, negative muon
Muons are elementary particles that are second-generation charged leptons in the Standard Model. They can have a positive or negative charge, both of which have a spin of 1/2 and a mean lifetime of 2.2 microseconds. Negative muons collapse into electrons, muon neutrinos, and electron antineutrinos by the weak interaction. Because negative muons are about 200 times heavier than electrons and are also negatively charged, they bind to positively charged nuclei and behave like "heavy electrons." Atoms composed of negative muons and nuclei are called muonic atoms. Muonic atoms eventually decay at the end of the life of the negative muon or when the negative muon is captured by the nucleus.
[3] Electronic X-ray
When orbital electrons on the inside of an atom (inner-shell electrons) are stripped for some reason, electrons from the outer orbits quickly fill the vacant orbits and any excess energy is emitted as X-rays. These X-rays are called electronic X-rays because they have more inherent energy than the atom. The electronic X-rays measured from the muonic iron atoms in this study were emitted when inner-shell electrons were stripped during muon deexcitation, and are also known as negative muonic X-rays.
[4] Quantum few-body system
A system composed of multiple particles that follows quantum mechanics. A muonic atom is a quantum few-body system composed of negative muons, multiple electrons, and a nucleus. These systems are difficult to handle theoretically because the different particles exert forces on each other and strongly interact.
[5] Materials and Life Science Experimental Facility (MLF), Japan Proton Accelerator Complex (J-PARC)
J-PARC is an accelerator facility in Tokai, Ibaraki Prefecture, where cutting-edge research using one of the highest intensity proton beams in the world is conducted in fields including elementary particles, atomic physics, materials, and life sciences. The muon experimental facility (MUSE) in J-PARC's Materials and Life Science Experimental Facility (MLF) is capable of generating the world's most intense muon beam.
[6] Bound muons, bound electrons
An electron that is spatially bound to the nucleus of an atom is called a bound electron. Its shell structure is based on its energy level, which are called the K shell, L shell, and M shell from inside to out. Similarly, negative muons that are bound to a nucleus are called bound muons. However, because their mass is about 200 times greater than that of an electron, the size of the shell structure is reduced by 1/200 and the bonding energy is 200 times higher.
[7] Excitation level, deexcitation
In quantum mechanics, muons and electrons bound to a nucleus must move in discrete orbits, and their energies are discretized (quantized) accordingly. When a particle is in the lowest-energy orbit, it is said to be in the ground level, and those in the higher-energy orbits are in an excitation level. Generally, the higher the energy level, the larger the orbital radius and the more unstable it is. The transition of a particle from a higher to a lower energy level is called deexcitation. With muonic atoms, the excess energy generated during deexcitation is mainly used to strip bound electrons.
[8] Full width at half maximum (FWHM)
This describes the width when a value is half of a certain observation value. With detectors, the smaller the FWHM, the higher the resolution of the observations. The TES used in this study has a FWHM of 5.2 eV, which far surpasses the resolution of conventional semiconductor detectors, which have a resolution of several hundred eV. This allowed the researchers to identify the structures of wide electronic K X-ray peaks.
[9] Kα X-rays, Kβ X-rays, hypersatellite Khα X-rays
Kα X-rays and Kβ X-rays are electronic X-rays emitted when a hole is opened in lowest energy ground level orbit and an electron from the surrounding area refills it. The energy of X-rays differs depending on which orbit the electron that fills the empty orbit comes from, and are called Kα X-rays and Kβ X-rays in ascending order of energy. Hypersatellite Khα X-rays are electronic X-rays that are emitted when two holes are created in the ground level orbit.
[10] Multiconfiguration Dirac-Fock method
A quantum mechanical method used to calculate the energy of multi-electron systems that takes the theory of relativity into account. By considering the interactions between various electron configurations, it is possible to calculate energy in a way that incorporates the correlations between electrons in quantum few-body systems.
* International research group
- RIKEN
- Atomic, Molecular, and Optical Physics Laboratory, RIKEN Cluster for Pioneering Research Research Fellow Takuma Okumura Head Researcher Toshiyuki Azuma Collaborative Researcher (during the study) Shinji Okada (currently associate professor at the Engineering Science Laboratory at the Chubu University College of Engineering) Basic Science Research Fellow (during the study) Yasuhiro Ueno
- Radioactive Isotope Physics Laboratory, RIKEN Nishina Center for Accelerator-Based Science Full-time Researcher Tadaaki Isobe
- U.S. National Institute of Standards and Technology (NIST)
Researcher Douglas A. Bennett Researcher William B. Doriese Researcher Malcolm S. Durkin Researcher Joseph W. Fowler Researcher Jonathan D. Gard Researcher Gene C. Hilton Researcher Kelsey M. Morgan Researcher Galen C. O'Neil Researcher Carl D. Reintsema Researcher Dan R. Schmidt Researcher Daniel S. Swetz Researcher Joel N. Ullom
- Kavli Institute for the Physics and Mathematics of the Universe (Kavli IPMU),
University of Tokyo Institute for Advanced Study Professor Tadayuki Takahashi Specially Appointed Assistant Professor Shinichiro Takeda Specially Appointed Researcher (during the study) Pietro Caradonna Specially Appointed Researcher Miho Katsuragawa Graduate student (during the study) Kairi Mine
- Graduate School of Science, Osaka University
- Department of Chemistry Assistant Professor (during the study) Kazuhiko Ninomiya Specially Appointed Researcher I-Huan Chiu
- Department of Earth and Space Science Assistant Professor Hirofumi Noda
- Japan Atomic Energy Agency (JAEA)
- Advanced Science Research Center Researcher Tadashi Hashimoto
- Materials Science Research Center Chief Researcher Takahito Osawa
- Department of Physics, Graduate School of Science, Tokyo Metropolitan University
Visiting Researcher Hideyuki Tatsuno Graduate Student Ryota Hayakawa Graduate Student Hirotaka Suda
- Department of Physics, Rikkyo University College of Science
Associate Professor Shinya Yamada Assistant Professor Yuto Ichinohe
- Kastler-Brossel Laboratory (France)
Professor Paul Indelicato Researcher Nancy Paul
- Institute of Materials Structure Science, High Energy Accelerator Research Organization (KEK)
Professor Koichiro Shimomura Specially Appointed Professor Yasuhiro Miyake Specially Appointed Associate Professor Naritoshi Kawamura Research Institute Lecturer Patrick Strasser Assistant Professor Sotaro Kanda Assistant Professor Satoshi Takeshita Researcher Motonobu Tanpo
- Fusion Systems Research Division, National Institute for Fusion Science (NIFS)
Associate Professor Taiji Kato
- Graduate School of Science, Tohoku University
Associate Professor Yasushi Kino Assistant Professor Kenichi Okutsu
- Department of Arts and Sciences, College of Liberal Arts, International Christian University
Professor Kenya Kubo
- Center for Computational Sciences, University of Tsukuba
Associate Professor Xiao-Min Tong
- Department of Space Astronomy and Astrophysics, Institute of Space and Astronautical Science (ISAS), Japan Aerospace Exploration Agency (JAXA)
Assistant Professor Shin Watanabe
This research was supported by Japan Society for the Promotion of Science (JSPS) Grants-in-Aid for Scientific Research on Innovative Areas "Toward new frontiers: encounter and synergy of state-of-the-art astronomical detectors and exotic quantum beams (principal investigator Tadayuki Takahashi)" and "Precise verification of atomic and molecular physics using negative muon beams and developments for astrophysical observation (principal investigator Toshiyuki Azuma)," Grants-in-Aid for Scientific Research (A) "Understanding the structure of thorium-229 nuclear isomers: new developments in high-precision chronological science (principal investigator Tadaaki Isobe)" and "Innovations in atomic and molecular dynamics research using superconducting molecular detectors (principal investigator Shinji Okada)," Grants-in-Aid for Young Scientists "Physical quantities of intra-cluster plasmas explored by precision X-ray spectroscopy observation and systematic exploration of microstructures (principal investigator Yuto Ichinohe)" and "Exploring chemical reactions of negative ions in cosmic environments using advanced neutral molecular detectors (principal investigator Takuma Okumura)", and the RIKEN Pioneering Project.