Sep 14, 2021PRESS RELEASE
Working toward precise measurements of the binding energy of hypernuclei
— Trying to unravel the hypertriton lifetime puzzle —
Keyword:RESEARCH
OBJECTIVE.
An international research group* used an originally developed machine learning [3] model to analyze data from a nuclear emulsion plate irradiated with a kaon [2] beam at the Japan Proton Accelerator Complex (J-PARC) [1], through which they visually detected the generation and decay of hypertritons [4], which are a type of hypernucleus [4]. The group included Senior Researcher Takehiko Saito of the High Energy Nuclear Physics Laboratory at the RIKEN Cluster for Pioneering Research, Senior Professor Kazuma Nakazawa of the Gifu University Graduate School of Engineering, Assistant Professor Junya Yoshida of the Tohoku University Department of Physics, and Associate Professor Masato Taki of the Rikkyo University Graduate School of Artificial Intelligence and Science.
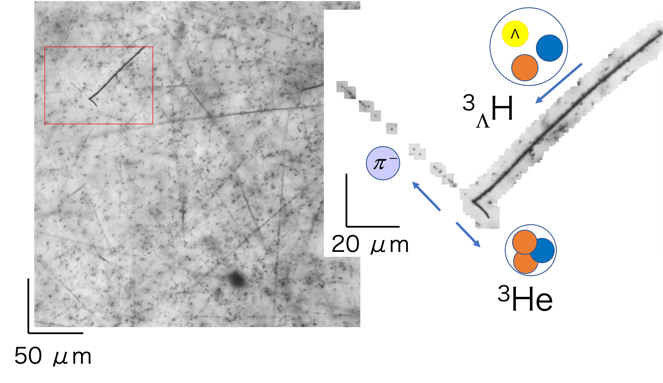
The decay of hypertritons the group visually detected
A hypertriton is composed of a deuterium nucleus [6] and a lambda (Λ) particle [7]. Measurements of the binding energy created from the forces acting between them obtained up to the 1970s have been trusted for about 50 years. However, recent experiments have shown that the lifetime of a hypertriton from formation to decay may be significantly shorter than the value predicted from its binding energy. Binding energy needs to be measured again precisely to resolve this contradiction, which is known as the hypertriton lifetime puzzle.
In this study, the international research group developed an analytical method that combines physical simulations and machine learning techniques, which they used to demonstrate that it is possible to detect the formation and decay of hypertritons from emulsion plate data.
This research was published in the online edition of the scientific journal Nature Reviews Physics on September 14.
* International research group
High Energy Nuclear Physics Laboratory, RIKEN Cluster for Pioneering Research
Basic Scientific Research Fellow Hiroyuki Ekawa
Graduate Student Research AssociateAyumi Kasagi
Senior Researcher (during the study) Nami Saito
Head Researcher Takehiko Saito
Researcher Yoshiki Tanaka
Trainee Wenbo Dou
Research Fellow Manami Nakagawa
International Program Associate Abdul Muneem
International Program Associate Enqiang Liu
Researcher He Wang
Graduate School of Engineering, Gifu University
Senior Professor Kazuma Nakazawa
(guest researcher, High Energy Nuclear Physics Laboratory, RIKEN Cluster for Pioneering Research)
Research Fellow (during the study)Masahiro Yoshimoto
Department of Physics, Tohoku University
Assistant Professor Junya Yoshida
(guest researcher, High Energy Nuclear Physics Laboratory, RIKEN Cluster for Pioneering Research)
Graduate School of Artificial Intelligence and Science, Rikkyo University
Associate Professor Masato Taki
Instituto de Estructura de la Materia (IEM), CSIC, Spain
Researcher Christophe Rappold
Research support
This study was supported by a Japan Society for the Promotion of Science (JSPS) grant-in-aid for scientific research (A) for "Understanding the interactions between lambda particles and between Xi baryons and nuclei (principal investigator: Kazuma Nakazawa)" and a grant-in-aid for new scientific research (proposed) on "Hierarchical structures of matter investigated with strange hadron clusters (principal investigator: Hirokazu Tamura)".
1. Background
A special atomic nucleus called a "hypernucleus" has been studied for many years to help understand the mechanism of the nuclear force. In addition to the protons and neutrons that make up a normal atomic nucleus, hypernuclei also contain particles called hyperons [7]. Protons and neutrons are composed of up quarks [8] and down quarks [8], respectively, while hyperons contain strange quarks [8]. By adding particles with properties that differ from protons and neutrons, it is possible to use hypernuclei to investigate how this changes the properties of atomic nuclei, which will broaden our framework of understanding of the nuclear force. Therefore, various hypernuclei with different combinations of protons, neutrons, and hyperons and with different types of hyperons have been created for study.
The lightest of the hypernuclei are the hypertritons, which contain a deuterium nucleus composed of a proton and neutron, and a lambda (Λ) particle, which is a type of hyperon. With these particles, there is what is known as the "hypertriton lifetime puzzle." This comes from the fact that experimental measurements of the lifetime of hypertritons from formation to decay are significantly shorter than the values predicted from the measurements of binding energy obtained up to 1970. In addition, lifetime measurements vary between experiments conducted in different parts of the world, making this an extremely difficult mystery to unravel. The WASA-FRS experiment led by principal investigator Takehiko Saito plans to measure the lifetime of hypertritons at the highest level of accuracy ever with a heavy ion accelerator in Germany in February 2022.
Measurements of the binding energy of hypertritons made using emulsion plates up to 1970 have been trusted for 50 years. However, a 2020 paper stated that binding energy measurements obtained with the STAR heavy ion collider at Brookhaven National Laboratory in the United States could be several times higher than those from 50 years ago *1). Yet this paper did not make a final conclusion due to large errors in the data, which only deepened the mystery surrounding hypertritons. To solve the hypertriton lifetime puzzle, not only their lifetimes need to be measured, but their binding energy must be re-examined at the highest level of accuracy possible.
*1) Nature Physics. 16, 409-412, DOI: 10.1038/s41567-020-0799-7 (2020).
2. Methods and findings
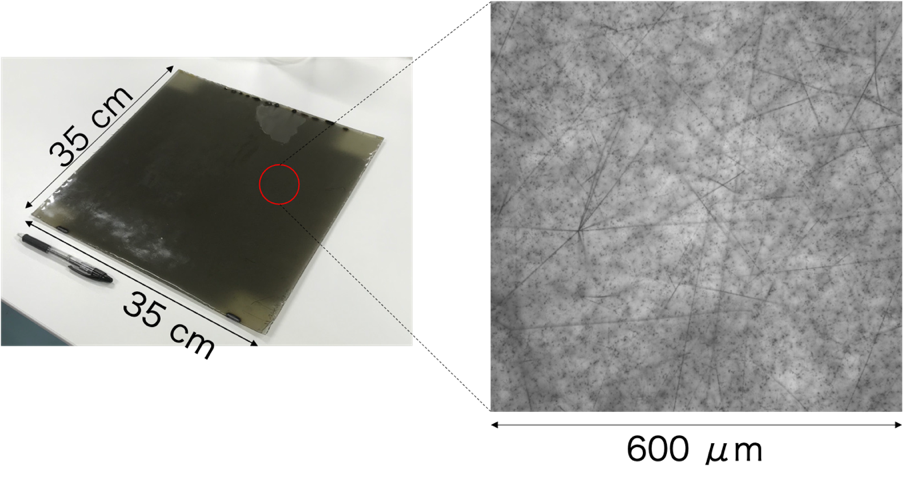
Figure 1. The emulsion plate used in the experiment (left) and an area of the plate enlarged using an optical microscope (right). The emulsion plate irradiated with a kaon beam at J-PARC (left) and an area of the plate enlarged using an optical microscope (right). The black lines are traces of charged particles called tracks. About 1 million tracks are recorded per square centimeter of emulsion plate.
However, because emulsion plates do not have time data, it is impossible to know when a particles passed through, and therefore the tracks of all particles that pass through a plate from immediately after it is manufactured to when it is developed are recorded as background events (Figure 1).
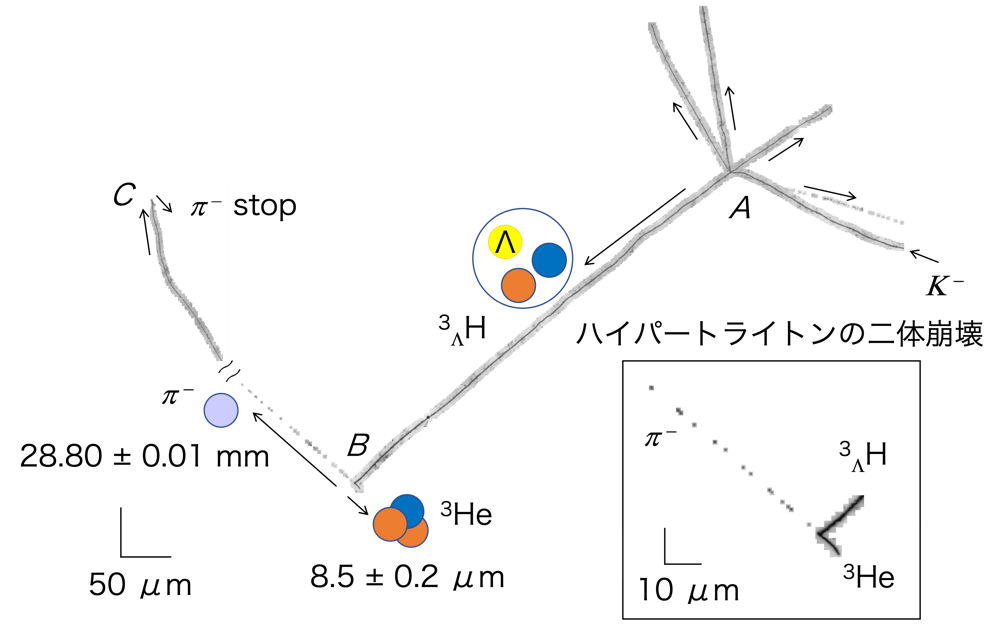
Figure 3. The first hypertriton event detected in this study A hypertriton (3ΛH) forms at point A in the emulsion plate, and after traveling to point B, it decays into a negative pi meson (π-) and helium-3 nucleus (3He). After traveling about 29 mm from where it was released at point B, the negative pi meson stopped at point C. The kinetic energy calculated from the length of its track and kinematic analysis identified it as a hypertriton event.
3. Future prospects
Analysis of the massive amount of data is ongoing, and the research group aims to solve the hypertriton lifetime puzzle through the most accurate measurements of hypertriton binding energy in the world and precise measurements of their lifetime in the WASA-FRS experiment.
This technique can be applied not only to hypertritons, but also to detecting other rare hypernuclear events. The emulsion plate currently being analyzed may contain records of undiscovered types of hypernuclei and of nuclei formation and decay events that have never been observed. To deepen our understanding of hypernuclei and the nuclear force through precise measurements of the bound energy of various hypernuclei and by detecting new events, further improvements and expansions to this technique are needed going forward.
4. Article information
Authors:
Takehiko R. Saito, Wenbou Dou, Vasyl Drozd, Hiroyuki Ekawa, Samuel Escrig, Yan He, Nasser Kalantar-Nayestanaki, Ayumi Kasagi, Myroslav Kavatsyuk, Enqiang Liu, Yue Ma, Shizu Minami, Abdul Muneem, Manami Nakagawa, Kazuma Nakazawa, Christophe Rappold, Nami Saito, Christoph Scheidenberger, Masato Taki, Yoshiki K. Tanaka, Junya Yoshida, Masahiro Yoshimoto, He Wang, Xiaohong Zhou
Journal: Nature Reviews Physics
<DOI>
10.1038/s42254-021-00371-w
5. Supplemental explanations
A complex that includes a high-intensity proton accelerator and other facilities in Tokai, Ibaraki Prefecture. The complex is jointly operated by the High Energy Accelerator Research Organization (KEK) and the Japan Atomic Energy Agency (JAEA). Secondary particles generated by colliding protons accelerated in the accelerator into atomic targets are used in research and industry in fields such as materials, life sciences, and nuclear and particle physics.
[2] Kaon
Mesons are particles composed of an equal number of quarks and antiquarks. Mesons that contain strange quarks are called kaons.
[3] Machine learning, neural network
Machine learning is a method of having a computer construct a processing method using large amounts of data with examples of correct answers (training data), rather than having a human program the processing method in advance. A neural network is a mathematical model used in machine learning that mimics the mechanism of the networks of neurons in living brains. Image processing using neural networks has rapidly improved since the mid-2010s, and is now applied in various situations.
[4] Hypernucleus, hypertriton
A hypernucleus is a nucleus that contains particles called hyperons in addition to the protons and neutrons that make up ordinary nuclei. A hypertriton is the lightest type of hypernucleus, and consists of protons, neutrons, and lambda particles (a type of hyperon).
[5] Binding energy
When an atomic nucleus and lambda particle bind to form a hypernucleus, the mass of the hypernucleus is less than the sum of the mass of the core nucleus and the mass of the lambda particle. This difference in mass is called the binding energy, and is a basic physical quantity of a hypernucleus. A hypertriton can be seen as a lambda particle bound to a deuterium nucleus consisting of one proton and one neutron, and its binding energy is defined as the mass of the hypertriton minus the masses of the deuterium nucleus and the lambda particle.
[6] Deuterium nucleus
The nucleus of a deuterium atom is composed of one proton and one neutron. Because the atomic number of an atom is determined by the number of protons, hydrogen atoms with one proton are 1H, while deuterium atoms are 2H.
[7] Lambda (Λ) particle, hyperon
The protons and neutrons that form ordinary nuclei are made up of only up quarks and down quarks, whereas particles containing strange quarks, which are the next heavier variety, are called hyperons. Hyperons include lambda (Λ) and sigma (Σ) particles, which contain one strange quark, xi (Ξ) particles that contain two, and omega (Ω) particles that contain three.
[8] Up quark, down quark, strange quark
Quarks are the basic particles that make up an atomic nucleus and are currently the smallest known unit of matter. Starting from the lightest, there are six types of quarks: up, down, strange, charm, bottom, and top. Each quark has an antiquark with approximately the same mass and an inverse charge.
[9] Mask R-CNN
A type of machine learning model used for object detection in images that was first reported in 2017. It is an accelerated and improved version of regions with convolutional neural network features (R-CNN), which is a type of convolutional neural network for object detection, that is capable of estimating the shape (mask) of the object to be detected.
[10] Negative pi meson
Among the pi mesons are the positive pi meson and negative pi meson, in which the particle that mediates the force that strongly binds protons and neutrons in the nucleus has a charge, and the neutral pi meson, in which this particle does not have a charge.
[11] Generative adversarial network (GAN)
A technique capable of generating more realistic simulated images in which learning takes place by having a neural network for converting input images into a desired style competes with a neural network for distinguishing whether the output image is real or fake.
6. Presenters, affiliations
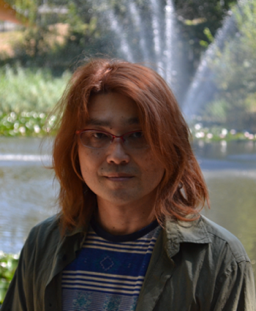
Takehiko Saito
High Energy Nuclear Physics Laboratory, RIKEN Cluster for Pioneering Research
Principal Investigator Takehiko Saito
Graduate School of Engineering, Gifu University
Senior Professor Kazuma Nakazawa
Department of Physics, Tohoku University
Assistant Professor Junya Yoshida
Graduate School of Artificial Intelligence and Science, Rikkyo University
Associate Professor Masato Taki
Inquiries
* RIKEN staff are working from home due to the novel coronavirus pandemic, so please make inquiries by email.
RIKEN, Public Relations Office, Press Officer
E-mail: ex-press[at]riken.jp
Gifu University, Administrative Department. General Affairs Division, Public Relations Section
TEL:058-293-3377 FAX:058-293-2021
E-mail:kohositu[at]gifu-u.ac.jp
Tohoku University, Graduate School of Science and Faculty of Science, Public Relations and Outreach Office
E-mail:sci-pr[at]mail.sci.tohoku.ac.jp
Rikkyo University, Public Relations Section
E-mail:koho[at]rikkyo.ac.jp
* Please replace [at] with @.