Sep 05, 2024PRESS RELEASE
Researchers discover mechanism of de novo membrane formation through membrane traffic rearrangement during gametogenesis
Keyword:RESEARCH
OBJECTIVE.
Researchers from Rikkyo University, the University of Tsukuba, and RIKEN have uncovered the mechanism of gametogenesis of budding yeast, a process involving meiosis and spore formation, by using live-cell imaging for detailed observation. In their study, the researchers discovered that both endoplasmic reticulum (ER) exit sites — which serve as the starting point for membrane traffic to transport essential proteins and lipids — and the Golgi apparatus are regenerated in nascent spores. This regeneration mechanism leads to the efficient formation of cell membranes of spores.
In the present study, live-cell imaging was employed to observe the process of meiosis and spore formation in detail, successfully capturing the moment when a membrane structure is formed inside a cell. The researchers focused on the secretory pathway which consists of the ER and the Golgi apparatus, transports proteins and lipids. They revealed that the number of ER exit sites, which serve as the starting point for membrane traffic, and the Golgi apparatus decrease during meiosis, but they reassemble during spore formation. The researchers’ search for molecules that control this process also led to the discovery of protein phosphatase-1 (PP-1) and its development-specific subunit, Gip1. They found that in cells lacking Gip1, ER exit sites were not regenerated, resulting in prospore membranes of abnormal size. In other words, during spore formation cells have a mechanism for remodeling the secretory pathway, which enables the efficient transport of lipids for prospore membrane formation.
Some diseases related to human gamete formation and fertilization are caused by failures in membrane traffic. The results of the present study are expected to elucidate the mechanisms by which these diseases develop, potentially leading to new diagnostic and treatment approaches.
Research representatives
- Assistant Professor Yasuyuki Suda
Faculty of Medicine, University of Tsukuba
- Professor Hiroyuki Tachikawa
College of Sport and Wellness, Rikkyo University
- Deputy Director Akihiko Nakano
Live Cell Super-Resolution Imaging Research Team, RIKEN Center for Advanced Photonics
Research background
Many proteins produced in a cell are transported through intracellular membrane traffic*1. ER and the Golgi apparatus are important organelles in this pathway. A wide variety of proteins and lipids produced in the ER, which are components of the cell membrane, are transported through specific regions of the ER membrane (ER exit sites) to the Golgi apparatus. The Golgi apparatus then sorts these proteins and lipids for delivery to their final destinations.
The life cycle of budding yeast, a eukaryote like humans (see Figure 1), includes the production of gametes through sexual reproduction. During meiosis and spore formation — gamete production in budding yeast — four new haploid cells, known as endospores, are produced inside a diploid cell (see Figure 2). In this process, new membrane structures are formed inside the diploid cell, enveloping the nucleus to create new cells, which are the spores. These spores will subsequently mature. However, it was unclear how membrane traffic, involving the ER and the Golgi apparatus, is controlled when supplying lipids to the new cell membranes at this stage.
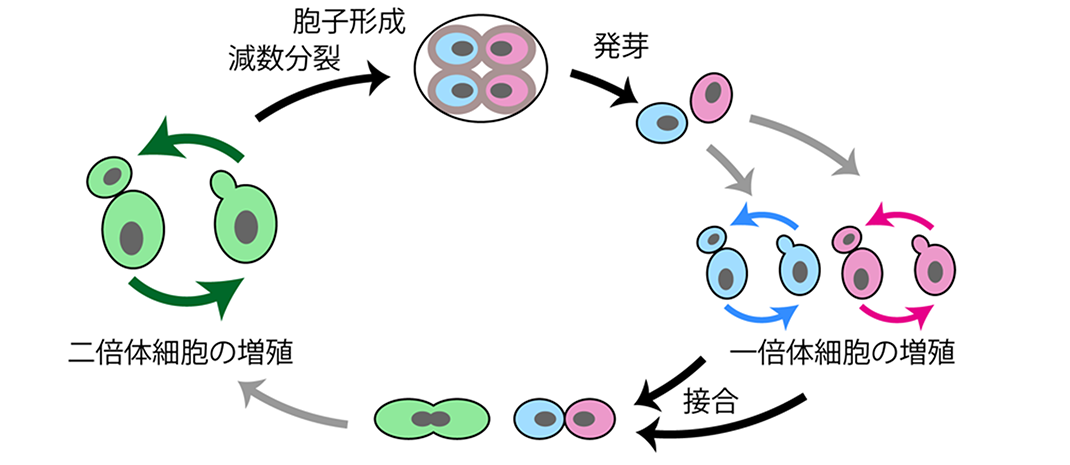
Figure 1 The life cycle of budding yeast. In budding yeast, haploid cells contain a single pair of chromosomes (indicated in blue and red), while diploid cells contain two pairs of chromosomes (indicated in green). Both haploid and diploid cells can exist stably, and proliferate through budding. When a haploid cell encounters another haploid cell of the opposite mating type, they conjugate to form a diploid cell. When a diploid cell experiences nutrient starvation, it undergoes meiosis to create spores. Under sufficient nutrient conditions, these spores can germinate and develop into haploid cells, restarting the proliferation cycle.
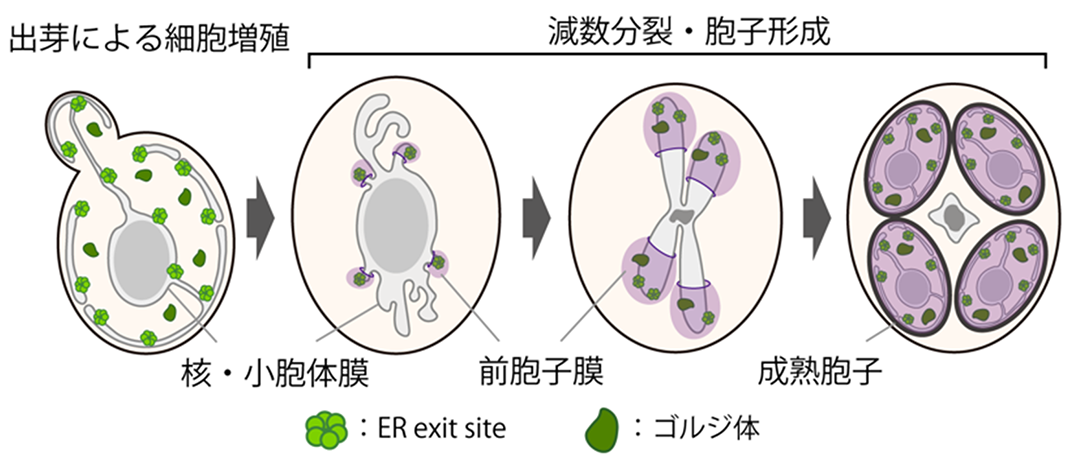
Figure 2 Formation of prospore membrane during meiosis and spore formation. The prospore membrane is formed within a cell during the middle stage of the second meiotic division, enveloping the nucleus and resulting in the production of mature endospores.
Research details and results
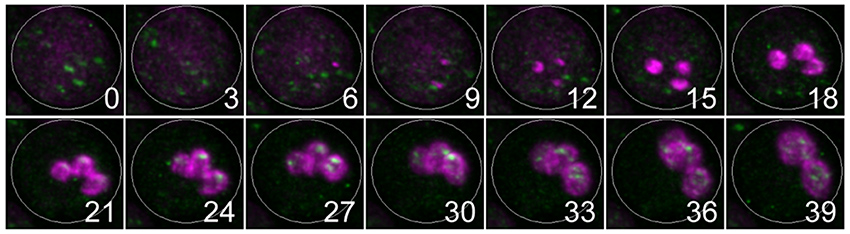
Figure 3 Prospore membrane formation and regeneration of ER exit sites. Using the SCLIM, the movements of prospore membranes (indicated in magenta) and ER exit sites (indicated in green) were observed. The numerals indicate elapsed time (minutes).
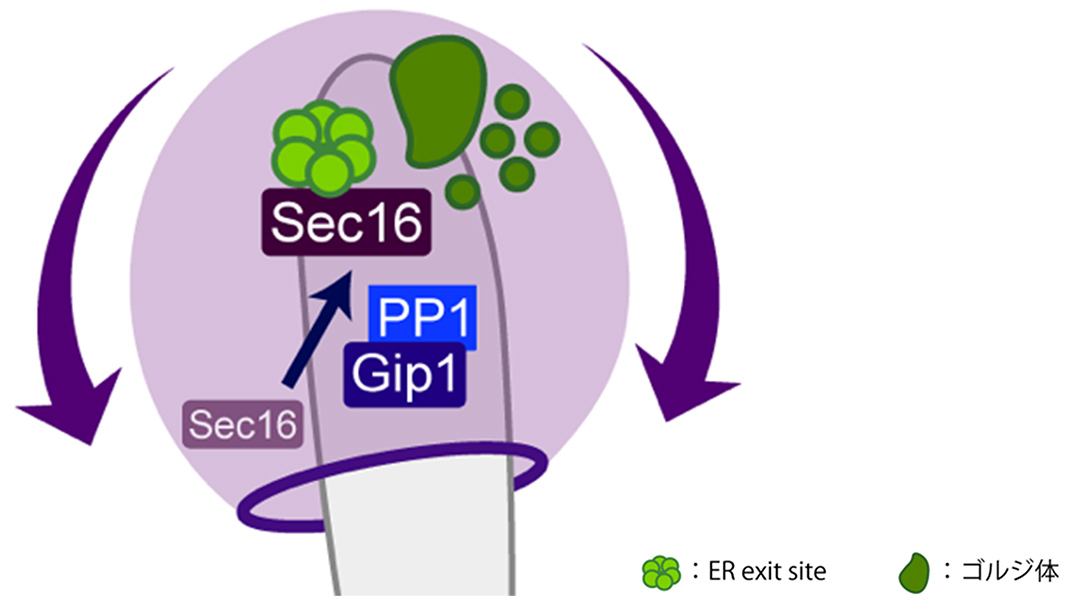
Figure 4 Model of prospore membrane growth. Protein phosphatase-1 and Gip1 work together to position Sec16 on the ER within the nascent spore, facilitating the regeneration of ER exit sites. The Golgi apparatus is subsequently formed to supply membrane lipids through the membrane traffic pathway to prospore membrane, enabling its proper growth.
Future prospects
Glossary
- *1: Membrane traffic
The system responsible for transporting proteins and other substances through vesicles and tubules between organelles in eukaryotic cells.
- *2: Live-cell imaging
A method that utilizes a microscopic system capable of capturing time-lapse images (frame-by-frame video) to observe changes in the movement and shape of cells and living organisms while they are alive.
- *3: Super-resolution Confocal Live Imaging Microscopy (SCLIM)
Developed by the Live Cell Super-Resolution Imaging Research Team at the RIKEN Center for Advanced Photonics, this microscopy system is ideal for observing and recording rapidly moving targets within cells.
Research funding
Published article
- Title: Remodeling of the secretory pathway is coordinated with de novo membrane formation in budding yeast gametogenesis
- Authors: Yasuyuki Suda (Assistant Professor, Department of Molecular Cell Biology, Faculty of Medicine, University of Tsukuba), Hiroyuki Tachikawa (Professor, College of Sport and Wellness, Rikkyo University), Tomomi Suda (Technician, Department of Molecular Cell Biology, Faculty of Medicine, University of Tsukuba), Kazuo Kurokawa (Senior Research Scientist, Live Cell Super-Resolution Imaging Research Team, RIKEN Center for Advanced Photonics), Akihiko Nakano (Deputy Director of RIKEN Center for Advanced Photonics), and Kenji Irie (Professor, Department of Molecular Cell Biology, Faculty of Medicine, University of Tsukuba)
- Journal: iScience
- Date of publication: August 29, 2024
- DOI: 10.1016/j.isci.2024.110855